Catalysis
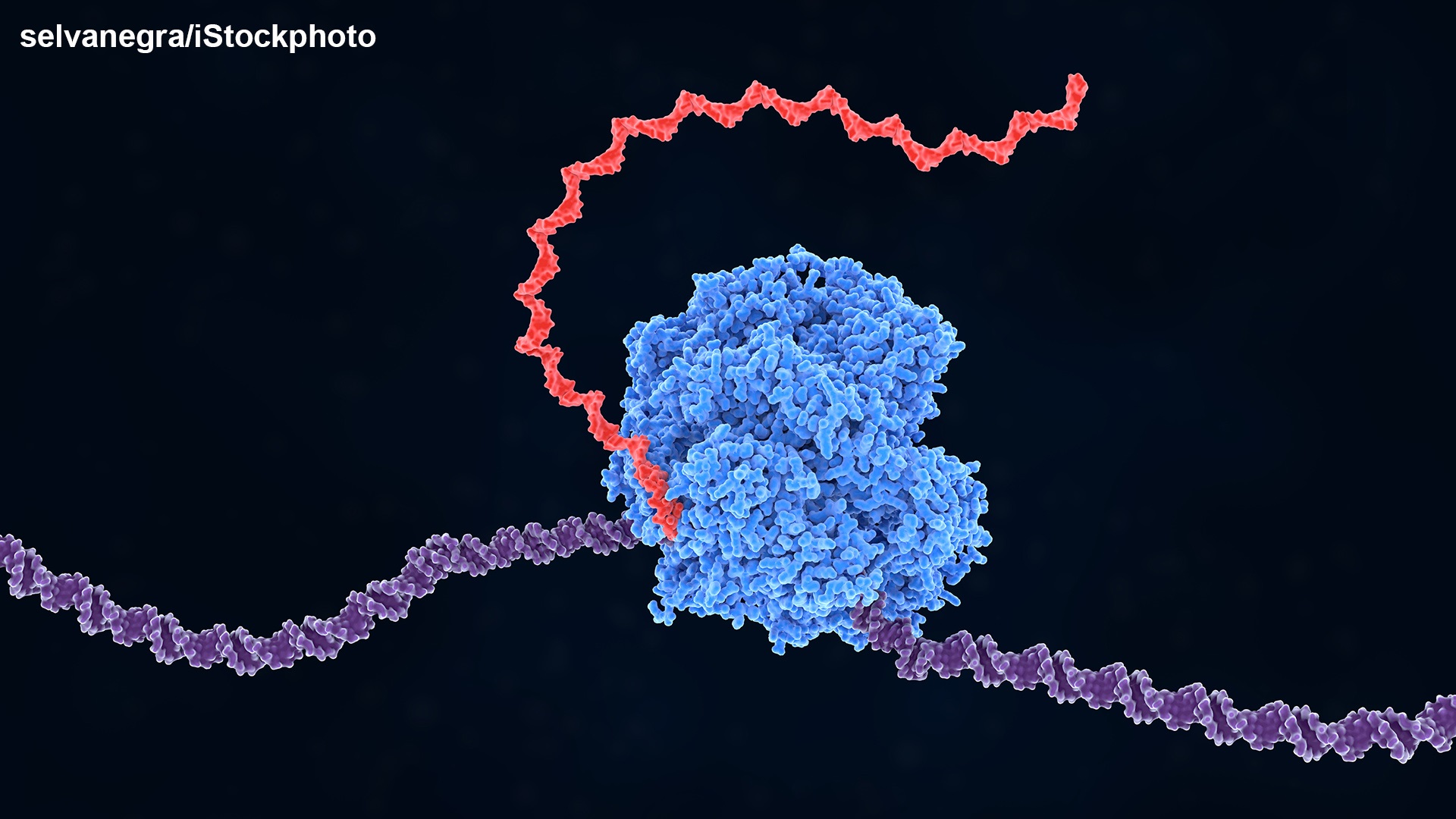
By Ben Skuse
The turn of the 20th century was an exciting time for anyone interested in science and technology. Max Planck (1918 Nobel Prize in Physics) was starting to unravel the quantum nature of energy, Guglielmo Marconi (1909 Nobel Prize in Physics) was hearing the first radio signal from across the Atlantic, Sigmund Freud was laying the foundations for psychoanalysis, and Felix Hoffmann was patenting aspirin. Hidden among all these headline-grabbing breakthroughs, a new field of study was germinating: catalysis.
Catalysis had been studied fitfully throughout the 19th Century. It was only when German chemist and philosopher Wilhelm Ostwald focused his efforts on the phenomenon that real progress was made in unravelling its nature. He systematically and quantitatively investigated catalysis and was the first person to recognise it as a kinetic phenomenon. In 1901, Ostwald summarised all that he had learned about catalysis in his paper ‘Über Katalyse’ and in September brought catalysis into the scientific mainstream at the meeting of the Gesellschaft Deutscher Naturforscher und Ärzte in Hamburg: “A catalyst is a material that changes the rate of a chemical reaction without appearing in the final product”, he said, likening it to oil in a machine, or a whip on a tired horse.
Catalysis Recognised
Months later, on a frigid December day in Stockholm in 1901, the first Nobel Prize in Chemistry went to Dutch physical chemist Jacobus Henricus van 't Hoff "for his discovery of the laws of chemical dynamics and osmotic pressure in solutions". Among many achievements in physical chemistry – including introducing the concept of chemical affinity, and correctly predicting the structures of allenes and cumulenes – van’t Hoff had a part to play in the embryonic stages of the science of catalysis. His eponymous equation linking chemical reactions to temperature forms the basis for the Arrhenius equation – the first equation to provide a quantitative description of catalytic reactions by Swedish polymath Svante Arrhenius (1903 Nobel Prize in Chemistry).
Just eight years later in 1909, the ‘father of catalysis’ Ostwald received the Nobel Prize in chemistry; well-deserved recognition for his numerous advances in the understanding of chemical catalysis, including the development of an important catalytic process, oxidating ammonia to make nitric acid in the Ostwald process.
(00:00:25 - 00:01:52)
Building on Ostwald’s foundations, French chemist and 1912 Nobel Prize in Chemistry recipient Paul Sabatier made important contributions to the science of catalysis. Not only did he discover a method to directly hydrogenate organic molecules using finely disintegrated nickel as a catalyst (forming the basis for margarine, oil hydrogenation and synthetic methanol industries), he also offered a kinetic understanding of the catalytic cycle and its corresponding elementary reaction steps through Sabatier’s principle. The principle provides a qualitative description of catalysis, highlighting that the binding energy between the catalyst and reactant should be neither too strong nor too weak. It has since been extended into a ubiquitous qualitative tool – density functional theory – to predict promising catalysts.
While theoretical advancements were beginning to increase our understanding of how catalysis works in the early 20th Century, many scientists were more interested in applying catalysts to create new chemical reactions in the real world. Alongside the earlier development of catalysts to produce key industrial substances like sulphuric acid and indigo, one of the most important advances was made in 1909 by German chemist Fritz Haber (1918 Nobel Prize in Chemistry), who proposed the synthesis of ammonia from nitrogen and hydrogen, using osmium as a new catalyst.
A tabletop experiment ill-suited to large-scale production, Haber approached Carl Bosch (1931 Nobel Prize in Chemistry) and BASF to scale up the process and find a suitable industrial catalyst. By exhaustive trial and error, Bosch managed to produce industrial-scale ammonia for the first time in 1913 using iron catalysis. The Haber–Bosch process, as it has become known, has been and continues to be pivotal to the expansion of the human population, with ammonia the raw material needed in the Ostwald process to make nitric acid – the basic ingredient of fertiliser nourishing the crops that feed the world. On average, half the nitrogen in a human body is the product of a Haber–Bosch plant.
Haber’s Nobel Prize award was highly controversial. He proposed the idea of releasing highly toxic chlorine gas into enemy trenches during World War I, essentially inventing chemical warfare, and he even supervised the first chlorine gas attack in Flanders, Belgium. However, the Nobel Committee concluded that his development of ammonia synthesis meant that he was a person who had given “the greatest benefit to mankind”.
(00:02:27 - 00:04:15)
Plastic Fantastic
Huge progress in applying catalysis in industry was made in the ensuing decades, with new catalysts producing a range of downstream chemicals. For example, the invention of catalytic cracking allowed the efficient processing of petroleum into fuels and chemicals.
Another important breakthrough, arguably defining 20th century life, was Ziegler–Natta polymerisation, independently invented in the mid-1950s and rewarded with the 1963 Nobel Prize in Chemistry for German chemist Karl Ziegler and Italian chemical engineer Giulio Natta. The innovative technique hinged on a group of catalysts – consisting of mixtures of transition metals halides like titanium, chromium, vanadium and zirconium, with organic derivatives of non-transition metals, particularly alkyl aluminium compounds – that enabled polymerisation at room temperature and normal atmospheric pressure, and exactly controlled the positioning of atoms attached to the polymer chains.
.
From this process, Natta eventually achieved the long-sought goal of creating a synthetic rubber molecularly identical to natural rubber. Most importantly though, the process made large-scale, high-quality polythene, polyethylene and polypropylene production possible, starting a plastics revolution that has seeped into almost every aspect of modern life.
(00:04:00 - 00:05:42)
(00:47:46 - 00:50:32)
Around the same time Ziegler and Natta were receiving their Prizes, surface chemistry – the study of the fundamental molecular processes at gas-solid interfaces – was gaining importance as an offshoot of the semiconductor industry. German physicist Gerhard Ertl (2007 Nobel Prize in Chemistry) was a pioneer in the field.
Inspired by a lecture from distinguished chemist Paul Emmett, Ertl decided to start applying surface science techniques to catalysis. He used new technology such as tunnelling microscopes to provide a complete picture of a surface reaction, first determining the molecular mechanism of ammonia synthesis in the Haber–Bosch process and later illuminating the oxidation of carbonmonoxide over palladium and platinum in catalytic converters.
(00:04:30 - 00:10:16)
A number of other key insights into catalysis have been rewarded in the 21st century by the Nobel Committee. In 2001, American chemists William Knowles and Barry Sharpless, and Japanese chemist Ryoji Noyori received the Prize for their work on chiral products between the late 1960s and 1980s. Chiral products derive from reactions that, under normal circumstances, produce an equal mixture of two products whose molecules are mirror images of each other, but can have very different properties. Each laureate developed chiral catalysts that tailor reactions so that only one of the two chiral molecules is produced.
Like Sabatier before them, Knowles and Noyori focused on hydrogenation, using transition metals to make chiral hydrogenation catalysts. Knowles’ research led to an industrial process for the production of the L-DOPA drug which is used in the treatment of Parkinson’s disease. Noyori developed this process further to today’s general chiral catalysts for hydrogenation, important within many industries, particularly in the production of safe pharmaceuticals. Sharpless, in contrast focused on oxidation. His methods have led to safer and more effective antibiotics, anti-inflammatory drugs, heart medicines and agricultural chemicals:
(00:11:20 - 00:17:42)
Another crucial advance in catalysis was made by French chemist Yves Chauvin, and American chemists Robert Grubbs and Richard Schrock, 2005 Nobel Prize in Chemistry recipients. These researchers developed catalysts for olefin metathesis, a chemical reaction that involves breaking and making double bonds between carbon atoms to produce new types of carbon–carbon double bonds. In 1971, Chauvin detailed how metathesis reactions function and what types of metal compound act as catalysts in the reactions. Two decades later, Grubbs and Schrock independently managed to produce efficient metathesis catalysts.
Olefin metathesis catalysts are now widely used in the chemical industry – mainly in the development of pharmaceuticals, but also fuels and advanced plastic materials – due to their synthesis methods being efficient, simple and environmentally friendly.
(00:00:50 - 00:06:46)
American chemist Richard Heck, and Japanese chemists Ei-ichi Negishi and Akira Suzuki shared the 2010 Nobel Prize in Chemistry for their work in the 1960s and 1970s in inventing “palladium-catalyzed cross couplings in organic synthesis”. Like Knowles, Sharpless and Noyori, these laureates focused on developing more precise methods of forming carbon–carbon bonds to produce organic molecules. They did so by coupling an electrophilic partner, such as an aryl halide or triflate, with a nucleophilic partner, such as an alkene or organometallic, using a palladium catalyst.
Today, the Heck reaction, Negishi reaction and Suzuki reaction are of considerable importance to chemists. Their reactions allow the production of sophisticated carbon-based molecules as complex as those created by nature itself, and are extensively used in research, and widely applied in the development and manufacture of many pharmaceuticals and high-tech materials.
(00:11:20 - 00:14:40)
(00:26:15 - 00:29:26)
Enzymatic Catalysis
Taking a broad view, every discovery mentioned up to now has been in developing metal-catalysed reactions. But despite its prominence and success, metal catalysis is not the only way to speed up a reaction. In fact, nature has been performing catalysis for aeons without any need for metals. Biocatalysis, or enzymatic catalysis, not only provides insight into biological processes but also produces a wide range of important products, from bread, cheese and beer to fine chemicals like amino acids and vitamins. Yet despite its ubiquity and importance, work on enzyme catalysis has only been rewarded by the Nobel Committee on three occasions.
The first Nobel Prize in Chemistry awarded for work on enzyme-catalysed reactions went to Australian–British chemist John Cornforth in 1975. In the 1950s, Cornforth exposed the three-dimensional structural aspects of enzyme-catalysed reactions. More specifically, he solved the details of the chemistry of the complex biosynthetic pathway in which acetic acid, containing two carbon atoms, is converted in nature into the steroid cholesterol, which contains 27 carbon atoms and has a structure containing four rings. His work paved the way for the development of modern cholesterol-lowering drugs and stimulated studies on the mechanism of a very large variety of enzyme-catalysed reactions, many of which are important targets in drug discovery.
(00:00:25 - 00:04:38)
The 1989 Nobel Prize in Chemistry went to Canadian–American molecular biologist Sidney Altman and American chemist Thomas Cech, who independently discovered that RNA weas more than simply an information transmitter to DNA. Between the 1970s and 1980s, they found that in certain conditions, RNA has catalytic properties too. A discovery that rewrote the textbooks, the functions previously attributed exclusively to protein enzymes were now known to also be performed by RNA called ribozymes.
Most recently, American chemical engineer Frances Arnold received the 2018 Nobel Prize in Chemistry “for the directed evolution of enzymes”. In 1993, she devised a method for inducing mutations in enzyme-producing bacteria and then screening and selecting the bacteria to catalyse and direct enzyme evolution. More specifically, Arnold directed the evolution of an enzyme called subtilisin so that it could break down milk protein casein in an organic solvent, dimethylformamide (DMF). Through iterative random mutations, in the third generation of subtilisin she found a variant that worked 256 times better in DMF than the original enzyme. Demonstrating the power of chance and directed selection to govern the development of new enzymes, directed enzyme evolution is now being wielded to synthesise new materials, biofuels, drugs and green plastics.
(00:06:46 - 00:11:21)
A Third Pillar
A relatively new form of catalysis – and often mistaken for biocatalysis – is organocatalysis, where a purely organic and metal-free small molecule is used to catalyse a chemical reaction. British chemist David MacMillan and German chemist Benjamin List were both awarded the 2021 Nobel Prize in Chemistry for their work on asymmetric organocatalysis.
Organocatalysts are chiral molecules that bind to the reacting molecules to form short-lived intermediates that are more reactive than the substrate molecules on their own. Being chiral, the catalyst transfers its handedness to the substrate, controlling which side of the intermediate can react further. In other words, organocatalysts can perform the same role as the metal catalysts developed by Knowles, Sharpless and Noyori, selecting the desirable version of a given chemical reaction’s chiral product.
The pair separately developed the technique in the 1990s. Up the then, the common wisdom among chemists was that a catalyst that synthesises chiral molecules had to either be an enzyme or contain a metal such as iron. List and MacMillan showed that small organic molecules can do the same job as big enzymes and metal catalysts in reactions that are precise, cheap, fast and environmentally friendly.
(00:08:06 - 00:11:23)
Catalysis for Green Chemistry
In recent decades, a strong focus in catalysis research has been on catalysing reactions that produce green fuels, reduce or eliminate hazardous waste, or help in some way to combat the effects of climate change. ‘Green chemistry’, as it is called, has been a passionate focus of laureates. Willard Libby (1960 Nobel Prize in Chemistry) may have received his 1960 Nobel Prize in Chemistry "for his method to use carbon-14 for age determination in archaeology, geology, geophysics, and other branches of science", but his 1974 Lindau lecture was on how heterogeneous catalysis can ameliorate the energy shortage.
(00:11:20 - 00:12:20)
Similarly, 1994 Nobel Prize in Chemistry recipient George Olah was awarded for ground-breaking work on superacids and carbocations, but devoted his first Lindau lecture in 1998 to carbon capture. Having investigated the topic in his lab, Olah proposed collecting carbon dioxide from factories and power plants and then subjecting it to catalytic processes to produce methanol. The yielded methanol could then be used for synthetic fuels, which could power vehicles even after Earth’s fossil fuel reserves run out.
(00:13:38 - 00:25:00)
Many of the laureates already mentioned also spoke passionately about catalysis and green chemistry. For example, Noyori emphasised the need to reduce the amount of undesired waste in the chemical industry, highlighting how waste can be minimised in his specialty of hydrogenation using molecular catalysts.
(00:07:57 - 00:22:27)
In 2013, Grubbs outlined how metathesis catalysis has been wielded in biorefineries to convert seed oils (instead of fossil fuel) to cost-effective, useful and environmentally friendly chemicals and fuels.
(00:06:48 - 00:11:49)
And most recently in 2020, Arnold presented some of her work harnessing directed enzyme evolution to produce cost-effective green biofuels and manufacture low-cost insect sex pheromones that disrupt pest mating and increase crop yields.
(00:11:44 - 00:25:36)
But the most insightful and wide-ranging insights into the role of catalysis in green chemistry have come from the many discussions on the topic over the years. A 2013 Panel debate featured Ertl, Grubbs, Schrock and a host of other laureates discussing the central role catalysis could play in, for example, hydrogen storage.
(00:03:50 - 00:09:10)
Schrock and Grubbs were again involved in 2021, giving their perspectives in an Agora Talk.
And the 2022 Meeting hosted a discussion between Schrock, MacMillan and young scientists. During this talk, emphasis was placed on using every tool in the catalysis scientists’ toolbox – metal catalysis, enzyme catalysis, organocatalysis and new ways to catalyse important chemical reactions – to ensure chemical products and processes reduce or eliminate the use or generation of hazardous substances.
“All forms of catalysis are really important for green chemistry,” said MacMillan. “We have what we already have, which is sustainable, but we need to keep inventing new forms of catalysis as well.”