Drug Targets
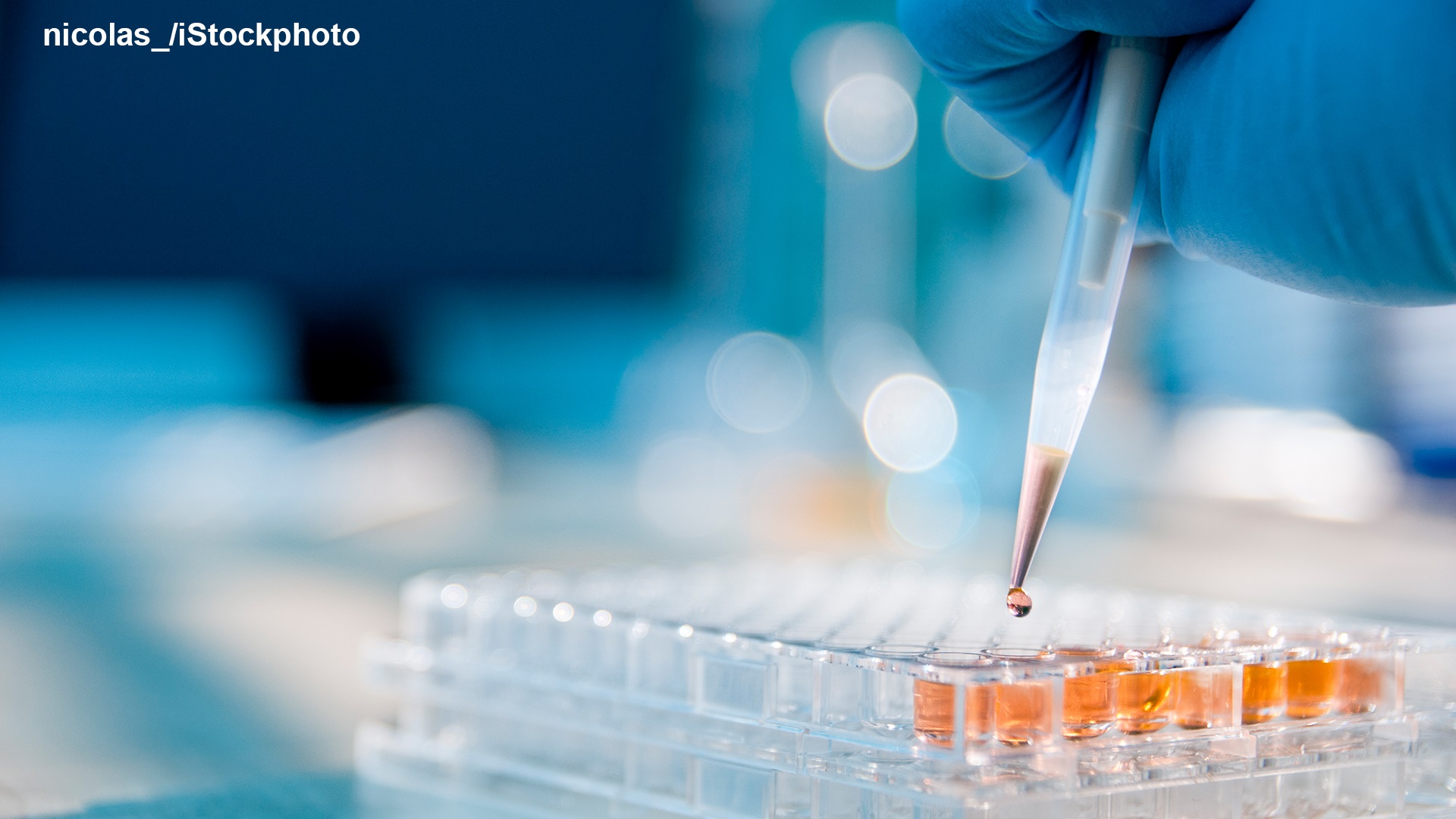
by Joachim Pietzsch
A Delayed Revelation
Most drugs have been known before their targets. Their development either built on traditional knowledge or they were discovered through specific observations at the bench or the bedside, often by chance or serendipity, while their mode of action initially remained obscure. Take acetylsalicylic acid for example.[1] Its chemical basis, salicylic acid, is contained in willow barks, which already were described as a source of medicines to treat fever and pain in Egyptian papyri more than 3000 years ago. In his “account of the success of the bark of the willow in the cure of agues”, the English chaplain Edward Stone revived this ancient knowledge in 1763. During the 19th century, salicylate medicines were quite popular in Europe despite of their adverse side effects. In 1897, the German chemist Felix Hoffmann succeeded in synthesizing a more tolerable derivative, acetylsalicylic acid. It was launched by Bayer two years later under the brand name aspirin and became one of the world’s best-selling drugs. The reason for its analgesic, antipyretic and anti-inflammatory effects was not revealed before 1971, however, when John Vane, the discoverer of prostacyclin, elegantly demonstrated that aspirin targets the enzyme cyclooxygenase (COX) and thereby inhibits the production of pro-inflammatory prostaglandins. This insight enabled the discovery of selective COX-2 inhibitors 20 years later. Together with Sune Bergström and Bengt Samuelsson, Vane shared the Nobel Prize in Physiology or Medicine in 1982 “for their discoveries concerning prostaglandins and related biologically active substances.” Some ten years before, together with the Brazilian pharmacologist Sérgio Ferreira, Vane had also discovered a new principle for the treatment of cardiovascular diseases and paved the way for the development of ACE inhibitors. Interestingly, the Karolinska Institute did not mention this achievement.
Four Nobel Prizes for Drug Innovation
While drug research arguably “has contributed more to the progress of medicine during the past century than any other scientific factor”,[2] only four Nobel Prizes in Physiology or Medicine have been explicitly awarded for advances in drug innovation in the 20th century: 1939 to Gerhard Domagk "for the discovery of the antibacterial effects of prontosil"; 1945 to Sir Alexander Fleming, Ernst Boris Chain and Sir Howard Walter Florey "for the discovery of penicillin and its curative effect in various infectious diseases"; 1952 to Selman A. Waksman "for his discovery of streptomycin, the first antibiotic effective against tuberculosis"; and 1988 to Sir James W. Black, Gertrude B. Elion and George H. Hitchings "for their discoveries of important principles for drug treatment". One could consider to include three other Nobel Prizes. Emil von Behring’s serum therapy, however, for which he was awarded with the first Nobel Prize in Physiology or Medicine in 1901, was a vaccination. Paul Ehrlich did in fact discover the first modern chemotherapeutic agent, salvarsan to treat syphilis - but he did so in the year after he shared the Nobel Prize with Ilya Mechnikov „in recognition of their work on immunity“ in 1908. And the discovery of insulin, which earned Frederick Banting and John Macleod the Nobel Prize in 1923, surely was a fundamental therapeutic breakthrough. Yet insulin is not a drug but a hormone.
Countless Contributions of Basic Research
In contrast, an almost countless number of Nobel Prizes in all three scientific disciplines has been awarded for inventions and discoveries of methods, structures and pathways that facilitated the identification of drug targets and the search for new drugs. The number of targets of current drugs is debated and estimated to lie between 218[3] and 483[4]. Their most prevalent biochemical classes are receptors and enzymes. Ion channels, transport proteins, nucleic acids and the ribosome offer additional targets. Growth factors, cell surface antigens, immunoglobulins and cytokines can be targeted by monoclonal antibodies. It would exceed the scope of this topic cluster to introduce all those Prizes in detail, so let us just have a selective and superficial look on them.
The Impact of Imaging
The most important methods for determining the structures of drug targets are X-ray crystallography and nuclear magnetic resonance. The development of the first originates in Röntgen’s discovery of X-rays (Nobel Prize in Physics 1901), is inaugurated by the discoveries of Max von Laue and William and Lawrence Bragg (Nobel Prize in Physics 1914 and 1915), advanced by techniques for the crystallization of enzymes, other proteins and viruses by James Sumner, John Northrop and Wendell Stanley (Nobel Prize in Chemistry 1946), and culminates in the structural elucidation of the first macromolecules by Dorothy Hodgkin (Nobel Prize in Chemistry 1964) and the first complex proteins by John Kendrew and Max Perutz (Nobel Prize in Chemistry 1962). Subsequently, Nobel Prizes related to X-ray crystallography were awarded both for methodological refinements (e.g. Nobel Prize in Chemistry 1985 to Herbert Hauptman and Jerome Karle) and the determination of numerous specific structures (from the Nobel Prize in Chemistry 1988 to Johann Deisenhofer, Robert Huber and Hartmut Michel “for the determination of the three-dimensional structure of a photosynthetic reaction centre" to the Nobel Prize in Chemistry 2012 to Robert Lefkowitz and Brian Kobilka „for studies of G-protein-coupled receptors). The development of nuclear magnetic resonance originates in the discoveries of Otto Stern and Isidor Rabi (Nobel Prize in Physics 1943 and 1944, respectively), and leads via the achievements of Felix Bloch and Edward Purcell (Nobel Prize in Physics 1952) and Richard Ernst (Nobel Prize in Chemistry 1991) to a Nobel Prize in Chemistry 2002 to Kurt Wüthrich „for his development of nuclear magnetic resonance spectroscopy for determining the three-dimensional structure of biological macromolecules in solution" and the Nobel Prize in Physiology or Medicine in 2003 to Paul Lauterbur and Sir Peter Mansfield „for their discoveries concerning magnetic resonance imaging".
The Power of Gene Technology
Without the invention of genetic engineering in 1973, drug researchers would not have been able to produce protein targets in sufficient amounts for high-throughput-screening and other preclinical assays nor would they be able to validate targets in knockout models. The importance of this tool is mainly reflected in the Nobel Prize in Physiology or Medicine 1978 to Werner Arber, Daniel Nathans and Hamilton Smith "for the discovery of restriction enzymes and their application to problems of molecular genetics", and in the Nobel Prize in Chemistry 1980 to Paul Berg "for his fundamental studies of the biochemistry of nucleic acids, with particular regard to recombinant-DNA" and to Walter Gilbert and Frederick Sanger "for their contributions concerning the determination of base sequences in nucleic acids". In Physiology or Medicine alone, 17 Nobel Prizes (1933, 1946, 1958, 1959, 1962, 1965, 1968, 1975, 1978, 1983, 1993, 1995, 2002, 2006, 2007, 2009, 2012) are associated with the preparation and further progress of gene technology, ranging from „discoveries concerning the role played by the chromosome in heredity“ (Thomas Morgan 1933) to “the discovery that mature cells can be reprogrammed to become pluripotent" (Sir John B. Gurdon and Shinya Yamanaka 2012), among them the Nobel Prize to Crick, Watson and Wilkins for unraveling the double helix structure of DNA (1962). Oswald Avery who had - against the prevailing view of his peers - identified DNA as the carrier of genetic information in 1943 did never receive a Nobel Prize, nor has the deciphering of the human genome in the year 2000 been awarded so far.
Pathways, Methods, Mechanisms
From the citric acid cyle to protein trafficking and vesicular traffic, from neurotransmission to immunology, from cellular signaling mechanisms to growth factors and oncogenes, from the patch-clamp-technique to the polymerase chain reaction: A plethora of discoveries and inventions that were rewarded with Nobel Prizes have spurred on drug discovery and target identification. To name but a few: The elucidation of the biosynthesis of cholesterol and the subsequent discovery of the LDL-receptor laid the ground for the development of HMG-CoA-inhibitors (statins), the world’s best-selling class of drugs (Nobel Prizes in Physiology or Medicine 1964 and 1985). The discovery that nitric oxide is identical with the endothelial derived relaxing factor and acts as an endogenous signaling molecule opened new horizons for the treatment of cardiovascular diseases – and, serendipitously, helped to develop drugs to treat erectile dysfunction (NP in Physiology or Medicine 1998). The discovery that reversible protein phosphorylation is a biological regulatory mechanism of outstanding importance (NP in Physiology or Medicine 1992) made kinase inhibition a fruitful field for drug development. Solving the structure of antibodies, understanding the creation of their diversity, and producing monoclonal antibodies were decisive steps on the way to today’s “biologicals” for the treatment of cancer and other diseases (NPs in Physiology or Medicine 1972, 1984 and 1987). The majority of currently available drug targets are G-protein coupled receptors (GPCRs). The Nobel Prizes in Physiology or Medicine 1971 (to Earl Sutherland who discovered the second messenger cAMP), 1994 (to Alfred Gilman and Martin Rodbell who discovered G-proteins and explained their role in signal transduction) and 2012 (to Robert Lefkovitz and Brian Kobilka who solved the structure of GPCRs) underline that significance.
Lindau Lectures on Drugs and their Targets 1951 - 2013
The development of drug discovery and the quest for novel targets is beautifully mirrored in lectures of many laureates in Lindau. Gerhard Domagk had discovered the first antibiotic chemotherapy, the sulpha drug prontosil, in 1932. From 1951 to 1963, the year before he died, he attended every Lindau Nobel Laureate Meeting. In the following lecture from 1960, he emphasizes the importance of this discovery by recalling how helpless medical doctors had been only three decades ago when confronted with bacterial infectious diseases: “We had to leave it to chance whether a patient succumbed to the disease or survived it.”
(00:07:15 - 00:09:42)
Selman Waksman who, shortly after the launch of penicillin, had discovered the first antibiotic remedy against the “white plague”, tuberculosis, in the 1940s, expressed himself even more enthusiastic about the impact of antibiotics. “This period, the last three decades, is known and will remain known as the golden age of antibiotics”, he said in his talk about “Successes and failures in search for antibiotics” in Lindau in 1969. “Some call it the atomic age, some the communications age, I prefer to call it the antibiotic age, because in no period of human history such blessings have been conferred upon mankind“. The development of resistance, however, had already become an issue and was discussed in Lindau both by Domagk and Waksman. Tuberculosis at least, he said, „is presently under complete control“. Bacterial infections obviously can be contained, but will this ever be possible for viral infections?
(00:33:35 - 00:36:16)
A Rational Road to Success
What appeared to be “the greatest waste of time and money” to Waksman, was a road to success for George Hitchings and Gertrude Elion. Right from the start of their 40-year-long collaboration, which was crowned by a Nobel Prize in 1988, they built their research on strategy rather than on serendipity. Their hypothesis was that it should be possible to selectively inhibit the synthesis of nucleic acids of bacteria, cancer cells and viruses without impeding the growth of normal cells. So they began to develop antagonists of nucleic acid bases – in times when the details of purine and pyrimidine synthesis had not yet been worked out. The results of this rational “antimetabolite approach” include inter alia the first treatment for leukemia, 6-mercaptopurine, the first immuno-suppressive agent, azathioprine, and the antiviral drug aciclovir for the treatment of herpes simplex, herpex zoster and varicella zoster infections. When Gertrude Elion came to Lindau in 1996, she thoroughly explained why the three compounds aciclovir, ganciclovir and azidothymidine act selectively on certain viruses. The latter was the first reverse transcriptase inhibitor for the treatment of AIDS.
(00:33:06 - 00:36:21)
With “a feeling of optimism that we can do something for AIDS patients that is more than we’ve been able to do in the past”, Elion concludes her talk and mentions the triple combination therapy. In the week after her Lindau lecture it was introduced in Vancouver and indeed proved to be the decisive turning point in the treatment of HIV infections.
Elion’s and Hitching’s co-recipient of the Nobel Prize, Sir James Black, who never lectured in Lindau, had pursued a different drug discovery strategy. He identified subtypes of certain receptors and developed drugs to inhibit them by molecular modeling. In 1964, he developed the first beta-blocker, propranolol, and eight years later he characterized the H2-subtype of histamine receptors, and subsequently developed the first H2-receptorantagonist – an effective medicinal treatment for patients with peptic ulcer who so far mostly had to undergo surgical treatment. The Japanese biochemist Akira Endo on the other hand never has been rewarded with a Nobel Prize nor did he receive any royalty for his breakthrough discovery that enabled the development of statins, which have generated cumulative sales of almost half a trillion dollars as of today. Statins inhibit excessive cholesterol synthesis and therefore are suited to treat certain cardiovascular diseases. They target the enzyme hydroxymethylglutaryl-CoA reductase, which catalyzes a key step in the biosynthesis of cholesterol. Konrad Bloch and Feodor Lynen, the two scientists who had earned the Nobel Prize in Physiology or Medicine 1964 for elucidating the complex biosynthesis of cholesterol, were frequent guests in Lindau. As a Nobel Laureate, Bloch had focused his research more and more on the role of lipids in the composition and function of cellular membranes, as his 1972 lecture shows:
(00:01:02 - 00:02:45)
Membranes, Channels and Receptors
Bloch’s interest was shared by Salvador Luria who was awarded with the Nobel Prize in Physiology or Medicine 1969 together with Delbrück and Hershey for their achievements in genetics but had discovered cellular membranes as an “unplowed field” he wanted to dig in already at the end of the 1950s. When he came to Lindau in 1981, he gave an interesting overview on different types of transmembrane channels:
(00:02:06 - 00:07:09)
The acetylcholine receptor to which Luria refers in this lecture, was of great interest for Sir Bernard Katz, too. He was a pioneer in solving the mechanisms of neuronal signaling, who was awarded the Nobel Prize in Physiology or Medicine 1970 jointly with Ulf von Euler and Julius Axelrod. Progress in synaptic cell physiology, he states in the following snippet from the lecture he gave in Lindau in 1978, has been mainly dependent on improvements in the methods of recording electrical signals:
(00:08:53 - 00:11:03)
The direct observation of single ion channels, which Katz mentions here, facilitated both basic research and drug discovery greatly. It had been enabled just two years before by the invention of the patch-clamp-technique by Erwin Neher and Bert Sakman whom Katz praises as if he already wanted to recommend them for the Nobel Prize in Physiology or Medicine, which they received in 1991.
Katz’ co-laureate Julius Axelrod (he had - without much recognition and no financial reward – discovered the painkiller paracetamol in his younger years) pointed out that acetylcholine receptors can be either ion channels or GPCRs in his Lindau lecture from 1993:
(00:17:09 - 00:18:49)
The surprising and fundamental discovery that reversible protein phosphorylation by kinases is one of the most widely used regulatory and signaling mechanisms of intracellular communication, was made by Edmond Fischer and Edwin Krebs who shared the Nobel Prize in Physiology or Medicine in 1992, already in the mid-50s. Fischer has been a regular speaker in Lindau. “I love this meeting and I wouldn't miss it for the world”, he opens this lecture from 2011, in which he introduces the ubiquity of protein phosphorylation and the large number of diseases in which it is involved:
(00:25:06 - 00:26:42)
The identification of nitric oxide as a messenger molecule, which is involved in the regulation of a large number of biological processes, has unraveled novel molecular targets and spurs on drug discovery and development not only in the field of cardiovascular diseases. For good reason, as Ferid Murad shows in his Lindau lecture from 2007, “the nitric oxide field is gone absolutely bananas” – from Murad’s first paper in 1977 to 82,000 publications thirty years later:
(00:45:14 - 00:48:05)
For his discoveries concerning nitric oxide as a signaling molecule, Murad shared the Nobel Prize in Physiology or Medicine 1998 with Robert Furchgott and Louis Ignarro. Eleven years later, Elizabeth Blackburn, Carol Greider and Jack Szostak received the Nobel Prize in Physiology or Medicine for the elucidation of a completely different but not less important physiological process. They had discovered that chromosomes are protected against degradation during cell division by telomeres. These caps on the ends of chromosomes are built by the enzyme telomerase. Telomeres delay the ageing of the cell, an effect that is desirable for healthy cells but not for cancer cells, which apparently escape senescence by preserving their telomeres. Therefore telomerase inhibitors one day might offer a new treatment option for some cancers, while on the other hand the stimulation of telomerase could offer new opportunities to treat degenerative diseases associated with ageing, including some other cancers. In her lecture in Lindau in 2011, Elizabeth Blackburn spoke about the potential therapeutic scope of her discovery:
(00:30:11 - 00:33:04)
Challenged by Unmet Expectations
Despite of promising discoveries of curiosity-driven basic research such as the ones of Blackburn and her co-laureates, however, the drug delivery pipelines of the pharmaceutical industry seem to have dried out since the turn of the century. The attrition rates in the process of drug development between target identification and the approval of a new drug have risen dramatically. The high hopes placed on the full sequencing of the human genome have not been fulfilled so far. Have the low-hanging fruits all been picked? Has the grace of serendipity left us? Are the complex processes of health and disease more complicated than anticipated? Why are we having trouble in making new drugs available for patients? Brian Kobilka, a medical doctor, who earned the Nobel Prize in Chemistry 2012 together with Robert Lefkowitz for their studies on GPCRs, shared his insights on this issue in his lecture in Lindau in 2013:
(00:05:50 - 00:13:01)
Many experts share Kobilka’s notion that the signaling behavior of receptors and their second messengers is too complex for a one target, one disease, and one drug approach. It may be necessary “to move away from the descriptions of single proteins, receptors and so on and to view the entire signal chain as the target”.[5] This approach may, for example, justify the success of the various multikinase inhibitors, which have amended traditional chemotherapies and considerably improved cancer treatment during the last decade. In the future, the success of this multi-target-approach will also depend on substantial input from systems biology. In times when “translational research” has become a mostly uncritically used buzz-word, it might perhaps also be advisable to remember the important role, which serendipity and careful clinical observations have played in the past: “In considering the historical perspective of drug discovery and the role of serendipity, it can be argued that the emphasis on translational research diverts scientists from pursuing basic-science studies that give rise to fundamental discoveries. In many cases, retro-translational research (from clinic to basic science) is necessary before the disease process can be understood well enough for scientists to develop therapeutics.”[6]
Footnotes:
[1] Cf. History of aspirin. (2014, May 31). In Wikipedia, The Free Encyclopedia. Retrieved 08:03, June 19, 2014, from http://en.wikipedia.org/w/index.php?title=History_of_aspirin&oldid=610986069
[2] Drews J. Drug discovery: a historical perspective. Science, 17 March 2000; 287: 1960 - 1963.
[3] Imming P, Sinning C and Meyer A. Drugs, their targets and the nature and number of drug targets. Nature Reviews Drug Discovery, October 2006; 5: 821-834.
[4] Drews J. l.c.
[5] Imming et al., l.c., p. 825.
[6] Fricker LD. Drug discovery over the past thirty years: Why aren’t there more new drugs? Einstein Journal of Biology & Medicine, March 2013; 29: 61-65, p.61.
Additional Lindau Lectures on Drugs and Their Targets:
1958: Hans von Euler-Chelpin „Chemische Struktur und biochemische Wirkungen“
1964: Linus Pauling „The structure of molecules in relation to medical problems“
1972: Feodor Lynen „Cholesterol and arteriosclerosis“
2007: Craig Mello „RNAi and development in C. elegans“
2011: Peter Agre „Aquaporin water channels: From atomic structure to malaria“
2013: Aaron Ciechanover „Drug development in the 21st century – Are we going to cure all diseases?“