Immunology
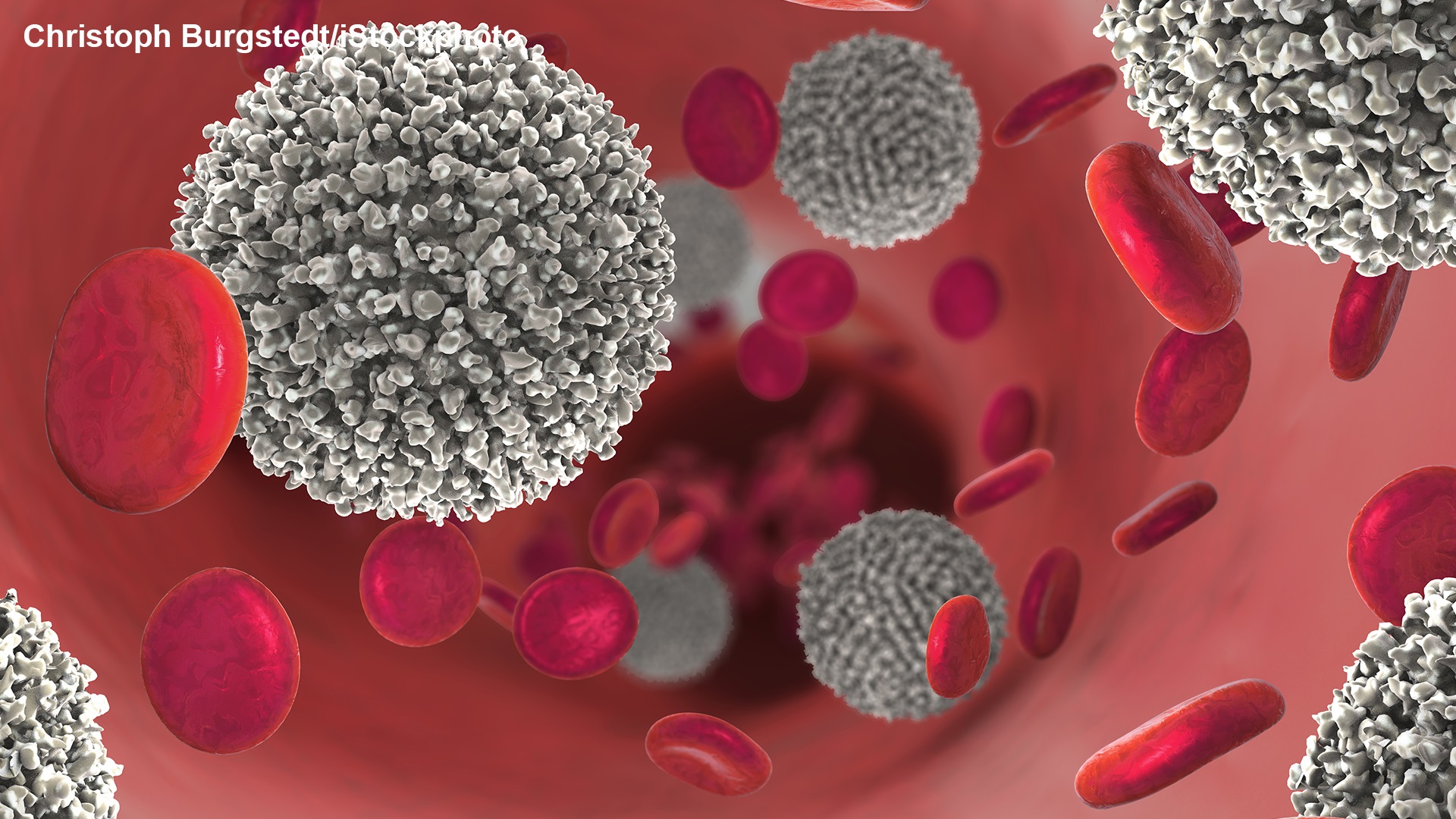
by Joachim Pietzsch
Biology or chemistry, that was the question, which accompanied immunology from its birth as a scientific discipline in 1883 all through the seven decades of its adolescence until 1957, and the dispute whether it is nobler to study cells or take advantage of molecules still lingers on today when immunologists ask whether a particular immune response is an innate or an adaptive one[1]. Gerald Edelman who shared the Nobel Prize in Physiology or Medicine 1972 with Rodney Porter “for their discoveries concerning the chemical structures of antibodies,” humorously illustrated this formative dichotomy of his discipline when he lectured in Lindau for the first time.
(00:00:36 - 00:01:31)
A formative dichotomy
Paul Ehrlich and Ilya Mechnikov shared the Nobel Prize in Physiology or Medicine 1908 “in recognition of their work on immunity”. It was the first Nobel Prize that in its quotation explicitly referred to immunity. For good reasons its award can be regarded as the official birthday of immunology.[2] It acknowledged the existence of two different arms of the immune system, a cellular defence line involving macrophages and a humoral defence line involving antibodies. By doing so, it reinforced the notion that “the phenomena of immunity (…) can be approached from various directions” and “that protection against disease can be of two kinds” [3].
In 1883, Ilya Mechnikov, a zoologist by training, had conducted an experiment, in which he introduced sharp splinters into transparent starfish larvae. The next day, he observed “a mass of moving cells surrounding the foreign bodies to form a thick cushion layer.” He deduced “that the afflux of mobile cells towards point of lesions” speaks for the hypothesis that “disease would be a fight between the morbid agent, the microbe from outside, and the mobile cells of the organism itself. Cure would come from the victory of the cells and immunity would be the sign of their acting sufficiently to prevent the microbial onslaught.” He succeeded in verifying this hypothesis in water-fleas. Consequently, he developed a general theory of immunity, which was primarily based on the action of “’phagocytes’, i.e. devouring cells, and the whole function that ensures immunity has been given the name of ‘phagocytosis’”.[4]
Emil von Behring, a medical doctor by training, and his colleague Shibasaburo Kitasato had taken a different approach to immunity. In their experiments, they observed that certain animals were not affected by infections with tetanus or diphtheria bacteria. They demonstrated that the administration of small doses of tetanus or diphtheria toxins stimulated the production of antitoxins in these animals, and that they were able to immunize other animals with these molecules. In December 1890, they published a paper, in which they proved the following hypothesis: “The immunity of rabbits and mice, which have been immunised against tetanus, is based on the ability of the cell-free blood liquid to render harmless the toxic compounds that are produced by tetanus bacilli”[5]. In a second paper, published one week later, von Behring added respective data on diphtheria. These two papers mark the beginning of serum therapy with anti-toxins, i.e., passive vaccination through the transfer of antibodies[6]. Clinical trials with a diphtheria antiserum that started three years later demonstrated an overall cure rate of almost 77 percent – a fantastic result in the fight against a disease that then was known as the “strangling angel of children”[7]. In 1901, von Behring received the first Nobel Prize in Physiology or Medicine “for his work on serum therapy, especially its application against diphtheria (…)”
Paul Ehrlich had been instrumental in realising von Behring’s success. He developed the methods for standardising antisera production and optimising its yield. In 1897, Ehrlich outlined his side-chain theory. He postulated that all cells of an organism carry a lot of preformed structures on their surface, which under healthy conditions “exist (…) in order to combine chemically with normal products of metabolism, i.e. to assimilate them”. Yet, in case of an infection and toxin injection, respectively, these structures could also bind bacterial toxins. “As these receptors, which may be regarded as lateral chains ("Seitenketten") of the protoplasm (…), become occupied by the toxin, the relevant normal function of this group is eliminated (…) - the deficiency is not merely exactly compensated, but made up to excess, i.e., there is hyperregeneration. Finally, if the injections are increased and repeated, so many such groupings are formed in the body of the cells that they inhibit as it were the normal functions and the cells get rid of the disturbing excess by discharging them into the blood.”[8] Although this theory proved to be wrong in many details, it offered the correct paradigm for the description of antibody formation and for our present understanding of adaptive immunity.
Chemistry takes over
Mechnikov’s cellular approach to immunity seemed not to be as applicable to medicine as humoral ones, in which pathogens could be defeated by antibodies, as von Behring and Ehrlich had proven. In the long term, it appeared that no infectious disease would resist the armamentarium of vaccination and serum treatment. Yet, such hopes did not fulfil. Consequently, immunologists shifted their focus towards chemistry and laid the emphasis of their research on antigens and antibodies, thereby inaugurating an era that lasted almost fifty years and was even dubbed “the ‘Dark Ages’ of immunochemistry.”[9] Nevertheless, three Nobel Prizes in Physiology or Medicine related to immunology were awarded during these years. To Charles Richet in 1913 “in recognition of his work on anaphylaxis”; to Jules Bordet in 1919 “for his discoveries relating to immunity”; and to Karl Landsteiner in 1930 “for his discovery of human blood groups.” The discoveries that lead to these Prizes had already been made between 1900 and 1902, however. Peter Medawar looked back at the era of the “old immunology”, as he called it, when he lectured in Lindau in 1978.
(00:01:27 - 00:03:09)
The chemists who dominated immunology tended to tune out the biological aspects of their discipline. “Allergy was left to the clinicians, and any excursions into questions of immunopathology were left to experimental pathologists, and little remarked in the mainstream of immunology.”[10] Yet several biological observations emerged, which could not easily be integrated into the prevailing chemical concept of immunology. These observations made it especially difficult to explain the huge diversity of antibodies. Their formation must be instructed by antigens, chemists argued. Antigens obviously serve as templates, which – comparable to casting a mould – determine the patterns of their respective antibodies. But how would an organism then be able to distinguish between self- and non-self-antigens to avoid autoimmune responses, and how could it be explained that antibody production eventually continues in the absence of antigen?
Return to biology
With his natural selection theory of antibody formation, Niels Jerne (Nobel Prize in Physiology or Medicine 1984) set out to answer such questions by returning to biology. According to Jerne, each higher organism possesses enough ready-made antibodies for all antigens it encounters. The enormous variety of antibodies is generated independently of foreign antigens. ”Somewhere in the beginning...we have to postulate a spontaneous production of globulin molecules of a great variety of random specificities in order to start the process. Possibly a specialized lymphoid tissue, such as that of the thymus...is engaged in this function”[11]. Because these antibodies developed in the foetus in the absence of external antigens they normally had acquired self-tolerance. Each antibody molecule selected the antigen with the best fit. As a result, the antibody would be shuttled into specialised cells for a stimulated production.
Jerne was almost right. Two years later, Frank Macfarlane Burnet went one step further, inspired both by Niels Jerne and David Talmage. “A modification of Jerne’s theory of antibody production using the concept of clonal selection” was the title of the paper he published in the „Australian Journal of Science“ on 21 October 1957. His intention was to optimise Jerne’s theory by ascribing “the recognition of foreign pattern (…) to clones of lymphatic cells and not to circulating antibody”[12]. As a result, he formulated his theory of clonal expansion: Lymphocytes express antibodies. Each lymphocyte carries only one antibody receptor. If it detects its suitable antigen, it begins to proliferate and thus produces a clone of countless lymphocytes that produce and secrete millions of antibodies against this specific antigen. When he came to Lindau in 1963, Burnet explained the essence of his theory to his audience:
(00:32:03 - 00:35:45)
The golden years of systemic immunology
The clonal selection theory can be regarded as a revolution. It “presented clearly for the first time the ideas that underlie the modern science of immunology”[13]. It reconciled the concepts of cell biology and humoral chemistry and established the basis for a mature, systemic immunology. When Burnet shared the Nobel Prize in Physiology or Medicine 1960 with Peter Medawar it was not for this theory, however, but for their “discovery of acquired immunological tolerance,” which had been proven in 1953. The ability to distinguish between “self” and “non-self” is a prerequisite for a normally functioning immune system. It also underlies the phenomenon of graft rejection after transplants of foreign tissues or organs. George Snell had begun to discover the body’s own antigens that are responsible for this rejection already in 1945 and first spotted the genes encoding them in 1951. Until the mid-1960s his work was complemented by Jean Dausset and Baruj Benacerraf. Consequently, the three scientists shared the Nobel Prize in Physiology or Medicine 1980 "for their discoveries concerning genetically determined structures on the cell surface that regulate immunological reactions". The early insights on self-recognition and graft rejection had challenged, as already foretold, the conventional instructive theories of antibody formation and finally spurred the formulation of the clonal selection theory.
After its publication, immunological research began to flourish in a broad variety. Within two decades (between 1959 and 1976) the main principles of the adaptive immune system were unravelled, resulting in the award of four Nobel Prizes in Physiology or Medicine: 1972 to Gerald M. Edelman and Rodney R. Porter "for their discoveries concerning the chemical structure of antibodies," 1984 to Niels K. Jerne, Georges J.F. Köhler and César Milstein "for theories concerning the specificity in development and control of the immune system and the discovery of the principle for production of monoclonal antibodies," 1987 to Susumu Tonegawa "for his discovery of the genetic principle for generation of antibody diversity," and 1996 to Peter C. Doherty and Rolf M. Zinkernagel "for their discoveries concerning the specificity of the cell mediated immune defence." Other very important results of research into adaptive immunity in those years included, to name but a few: 1961 the discovery of the role of the thymus for the development of the immune system by Jacques Miller, 1962 the proof that lymphocytes are cells that circulate between blood, tissue and lymph as potential initiators of the immune response by James Gowans, and 1965 the identification of two separate lymphocyte lineages, namely T and B cells, by Max Cooper.
Within these golden years of systemic immunology, in 1973, Ralph Steinman discovered the dendritic cell, which he later showed to have an exceptional capacity to activate T lymphocytes. Thus, dendritic cells turned out to be the crucial link between the innate and the adaptive immune system. How the innate immune system is activated, however, remained unknown until the late 1990s when Jules Hoffmann and Bruce Beutler answered this question. "For their discoveries concerning the activation of innate immunity” they shared one half of the Nobel Prize in Physiology or Medicine 2011. The other half went to Ralph M. Steinman "for his discovery of the dendritic cell and its role in adaptive immunity." Sadly, Ralph Steinman passed away before the news of his Nobel Prize reached him.
Two immune response-abilities
Eleven of the 15 laureates who have received a Nobel Prize related to immunology between 1960 and 2011 have lectured in Lindau. Their lectures offer an excellent source to briefly summarise our present knowledge on what happens when a pathogen attacks our body.[14] Any pathogen is a harmful invader. Yet not every microbe is a pathogen. To the contrary, most microbial species are useful companions of our life on earth. Even when they reside within our body, they are normally good friends without whom we couldn’t live. In fact, each of us consists of more microbes than proprietary cells. Our body contains about ten trillion human cells while it is additionally colonised by a hundred trillion bacterial, fungal and protozoan cells and even viruses whose entirety represents our normal flora[15]. Today we know that an imbalance in this microbial flora may contribute to many diseases. Its constituents are harmless, however. Pathogens, on the other hand, are dangerous. They surround us anywhere at any time. We eat them, we drink them, we inhale them. They sit on our skin or mingle with our mucosa. Thanks to our immune system, however, most of them fail in making us sick. This system protects us with two separate but intertwined defence mechanisms. Innate immune responses (to which Mechnikov’s phagocytes belong) appeared already in the first multicellular organisms one billion years ago, whereas additional adaptive immune responses (to which Ehrlich’s antibodies belong) began to emerge in vertebrates about 450 million years ago, as Jules Hoffmann illustrated in the summarising slide of his lecture in Lindau 2014.
(00:25:16 - 00:26:03)
The triggers of innate immunity
When a pathogen tries to attack us, it mostly fails at the epithelial surfaces that form our skin or that line the mucosa of our respiratory, digestive, urinary and digestive tract. These surfaces, which cover the impressive area of about 100 m2 in our respiratory and up to 300 m2 in our digestive tract serve as both physical and chemical barriers[16]. If a pathogen nevertheless breaches one of these barriers of our body, non-epithelial cells of the innate immune system come into play and strive to render the invader harmless. It is important to note that this confrontation in most cases takes place in our tissue. A pathogen should never reach our blood circulation, otherwise a fatal sepsis could be the consequence. First of all, macrophages that reside within the tissue appear at the site of the invasion, followed by neutrophils that migrate from the blood. But how do these cells detect the presence of a pathogen? They can sense pathogen-specific molecules that normally are not present in our body, the so-called pathogen-associated molecular patterns (PAMPs) through their pattern recognition receptors (PRRs). The first PRR was discovered by Jules Hoffmann in 1996 when he studied how fruit flies fight fungal infections. He made his breakthrough discovery of a specific receptor complex involved in activation of innate immunity in a fruit fly with a specific gene mutation, which its originator Christiane Nüsslein-Volhard (NP in Physiology or Medicine 1995) had called “toll” – the German word for “great” or “fantastic”. Hoffmann concluded that the “toll” receptor was necessary for fruit flies to be able to cope with fungal infection. When he came to Lindau in 2014, he spoke about the publication of his seminal paper and its consequences.
(00:09:05 - 00:12:59)
When Jules Hoffmann discovered the Toll receptor in the fruit fly, Bruce Beutler had already been working for several years on a seemingly unrelated topic, namely how exactly the lipopolysaccharide (LPS) of Gram-negative bacteria induces septic shock. Beutler suspected that a specific receptor for LPS existed. In search of this receptor, he systematically screened the genome of mouse mutants that could not react to LPS. He discovered that they had a mutation in a gene, which resembled the fruit fly’s Toll gene and encoded for the Toll-like receptor (TLR)-4. Thereby he confirmed that insects and mammals share a molecular sensor system, which activates the innate immune system. In 2015, he shared the excitement of this discovery with his audience in Lindau.
(00:09:25 - 00:11:56)
Besides toll-like receptors, several other PRR families exist. Their mode of action is principally the same. Once a PRR has bound a PAMP, it triggers the secretion of signal molecules. This leads to a vasodilation, enabling further phagocytes to approach the site of infection and to induce a local inflammation. An acute inflammation is a good sign for a healthy immune response. It shows that the macrophages and neutrophils summoned to fight the infection are busy destroying pathogens.
Parasites that are too large to be ingested are surrounded by a group of phagocytes and killed during a joint attack with toxic molecules. Viral infections, however, are not associated with PAMPs. The host cell, after all, has been forced by the virus to produce its proteins. PRRs therefore strive to detect other suspicious molecules of a virus. If they succeed, they secrete specialised cytokines (type I interferons), which in turn can recruit natural killer cells that force the virus-infected cells to commit suicide by apoptosis.
A humoral cascade and a cellular mediator
The innate immune system does not rely on cellular responses alone, though. It also has at its disposal a powerful humoral defence, namely the complement system, which principally has already been discovered at the end of the 19th century by Hans Buchner and Jules Bordet (NP in Physiology or Medicine 1919). The complement system is an amplifying proteolytic cascade. It consists of 30 interacting soluble proteins. Its activation ultimately causes the formation of a protein complex on the surface of a pathogen. This complex pierces aqueous pores in the pathogen’s membrane to destroy it. An insight into the functionality of the complement system was given by Rodney Porter in Lindau in 1984:
(00:06:58 - 00:10:04)
In many cases, such as after a scrape for example, the joined forces of phagocytes and the complement cascade will suffice to eliminate all invading pathogens, and the immune response ends. If this is not the case, the adaptive immune system is employed. This employment needs to be mediated by professional antigen-presenting cells of whom dendritic cells are the most potent ones. Dendritic cells were discovered by Ralph Steinman in the 1970s. They continuously patrol our tissues. When they detect a microbial invader, they engulf it and cleave it into peptide fragments. Then they migrate to a lymph node, where they present these fragments to T cells and activate them. Dendritic cells are also able to inhibit T cells, when it is necessary to avoid an overshooting immune reaction.
The two main actors of adaptive immunity
Our innate immune system reacts immediately once it has been activated by a pathogen. Its response lasts no longer than four days approximately and retains no memory of the invader. It is very effective against bacteria while disposing of modest means against viral infections. Our adaptive immune system, by contrast, reacts delayed in time. Normally, it cannot be directly activated by a pathogen. It rather depends on mediation of the innate immune system to be called into play. It disposes of a powerful armamentarium against viral infections. It develops a memory of its pathogenic enemies so that it can react more quickly at re-infection and is able to develop long-lasting immunity against established invaders.
The power of our adaptive immune system is based on the smart and sophisticated actions of two kinds of lymphocytes, which are called B cells and T cells, and whose entire cell mass resembles that of our brain. B cells develop in the bone marrow and T cells develop in the thymus. Their activation takes place in peripheral lymphoid organs such as the lymph nodes, the spleen and epithelium-associated lymphoid tissues. T cells are activated by dendritic cells, B cells either by antigen (plus innate signals) or with the help of T cells.
Activated B cells are called plasma cells. They can express five different classes of proteins that belong to the group of immunoglobulins (Igs). They both incorporate Igs into their cell membrane as B-cell receptors (BCRs) and secrete them as antibodies into the extracellular space. These antibodies fulfill two main functions: they neutralise extracellular viruses and toxins by preventing them to bind to receptors of potential host cells; and they mark pathogens for destruction by components of the innate immune system. T cells appear in three main classes: cytotoxic T cells destroy intracellular viruses by killing the virus-infected cells; helper T cells stimulate the activity of other cells of the innate and adaptive immune system while regulatory T cells serve as suppressors of those other immune cells. With their antibodies, activated B cells have a large operating range; activated T cells, in contrast, mainly act at short range. In the lecture he gave in Lindau in 2014, Rolf Zinkernagel emphasised the importance of a well-balanced interplay of B and T cells.
(00:18:26 - 00:20:26)
Setting two stages for effecting T cells
Antibodies detect and catch antigens that exactly fit their form. T cells can recognise antigens only if the host cell has processed these antigens into peptide fragments and presents them on its surface. The structures that display these antigen fragments are called MHC receptors. MHC stands for major histocompatibility complex. Each cell of our body displays MHC receptors. They resemble molecular identity cards of our body’s own tissue and are involved in the rejection of foreign tissue. Originally, they were discovered in transplantation research, where they became known as human leukocyte-associated (HLA) proteins. Jean Dausset was able to show that these HLA proteins are encoded by a single genetic complex system on one single chromosome. Four years after he had been awarded a Nobel Prize for this achievement, he lectured in Lindau.
(00:02:52 - 00:04:38)
The insight that MHC receptors are not only involved in the rejection of foreign graft but also play a decisive role in the normal immune response of our body was gained by Peter Doherty and Rolf Zinkernagel in 1974. They conducted experiments with mice to study how the animals defended themselves against a viral infection. As expected, they observed that the mice developed cytotoxic T cells, which could kill virus-infected cells of these mice in a test tube. Unexpectedly, however, these T cells were not able to kill infected cells from another strain of mice. Apparently, the viral antigen alone could not provoke an effective T cell response. Additionally, the host organism’s proper MHC receptor had to be in place. Only if a viral antigen is presented by a MHC receptor, the infected cell will be recognised by a T cell.
During its development, each lymphocyte, both of B and T origin, is trained to recognise and respond to one specific antigen only. After being released from its “training camp” in the bone marrow or the thymus, each lymphocyte is continuously circulating in the blood and lymph stream until it encounters its specific antigen (or is replaced by its successor). Upon binding this antigen for the first time, it proliferates and produces a huge number of cells with the same specificity – the process of clonal expansion takes place, just as Burnet had hypothesised. At the same time, the cells of that clone differentiate into effector cells and memory cells. The latter are our body’s reservoir for a stronger and faster secondary immune response upon re-infection months or years later. The former move into the ongoing battle. How effectively they fight was shown by Peter Doherty in his lecture in Lindau in 2015, in which he also referred to the discovery of MHC restriction.
(00:16:50 - 00:19:15)
Y antibodies are very diverse
The structure of antibodies – elucidated by Gerald Edelman and Rodney Porter – has become an icon of molecular biology, second only to the double helix. Its Y-shaped form, made up of two identical heavy and two identical light polypeptide chains, almost suggests its function, too. The N-terminal regions of the light and heavy chains with their variable amino acid sequences form the antigen-binding sites of an antibody. There are five types of heavy and two types of light chains. The C-terminal regions of an antibody’s heavy chains form its tail or Fc region, which can be involved in the activation both of the complement and the cells of the innate immune system.
The variable regions of the heavy and light chains of an antibody are encoded for by three and two different gene segments, respectively, which are located on three different chromosomes. Their rearrangement and recombination hold the clue for the almost limitless diversity of antibodies, enabling them to detect virtually any antigen. A developing B cell joins together these different segments. Then it draws at random genes from each of them. This can be compared to a lottery where numbers are drawn from different pots. The drawn genes finally encode for the respective antibody’s specific antigen-binding site. This gives rise to huge combinatorial possibilities. A loss or gain of nucleotides at the site of the joining further increases this number. Additionally, after antigen stimulation, somatic mutations occur that gradually increase the affinity of an antibody to its antigen during the course of an infection. Susumu Tonegewa who solved the puzzle of combinatorial diversification, explained this process precisely in Lindau.
(00:09:38 - 00:12:37)
An extraordinary valuable invention
The extraordinary capabilities of antibodies nourished the dream that it would become possible to produce monoclonal antibodies. In 1975, César Milstein and his post-doc Georges Köhler succeeded in making this dream come true. They fused a mutant cell line of mouse myeloma (a cancer of plasma cells) with mouse spleen cells that had been immunised with erythrocytes from sheep. They incubated them in a specific medium, in which only fused cells could survive. Thereby, they created a hybridoma with the immortality of a tumour cell and the specific ability to produce antibodies against sheep erythrocytes. The importance of their invention was not immediately realised by the scientific community. Nature didn’t accept their paper to be published in “the section reserved for findings of leading importance” but only accepted is as a letter to the editors. Gradually, however, the enormous impact of Milstein’s and Köhler’s invention became clear. “Besides gene technology, which has already been honoured by several Nobel Prizes, the hybridoma technique represents the most important methodological advance within the field of biomedicine during the 1970s,”[17] the Karolinska Institute stated on occasion of the award of the Nobel Prize in honour of “the principle for production of monoclonal antibodies.” Soon, monoclonal antibodies became a powerful tool in research and diagnostics. For therapeutic use, though, they had to be humanised, because antibodies generated in rodents are immunogenic. As soon as this difficult problem had been solved, the triumphal procession of monoclonal antibodies in clinical medicine began, mainly as therapies for autoimmune diseases and cancer. As of 2015, almost 30 monoclonal antibodies had received regulatory approval by the European Medical Agency[18]. “Such cultures could be valuable for medical and industrial use,”[19] was the last sentence of Köhler’s and Milstein’s letter to Nature in 1975. Forty years later, the global antibody market was estimated at 75 billion US-Dollars[20].
Sadly, Georges Köhler didn’t live to see this success. He passed away in 1995. In Lindau, he did not talk about his invention. “It’s a technique, which is now well-known, and the story is, for me, over,” he modestly opened his lecture in 1990, in which he went on to describe why transgenic mice are important and indispensable in immunological research.
(00:12:46 - 00:16:04)
The foreignness of tumour cells
As mentioned before, the ability to distinguish self from non-self is the fundamental prerequisite for a normal function of our body’s immune defence, which both B and T cells need to learn during their development. A failure in this distinction can lead to severe autoimmune diseases, in which or body attacks its own cells. On the other hand, immune cells must not react to harmless, non-pathogenic foreign substances. Otherwise, severe allergic reactions may occur. To enable tissue and organ transplantations, our normally healthy hostility against foreign antigens has to suppressed. In his lecture in Lindau in 1978, Peter Medawar, one of the pioneers of transplantation medicine, looked back at his work in this field – and established its connection with the fight against cancer.
(00:07:31 - 00:08:56)
Today, we know that Medawar’s observation “that some cancers arouse a defensive rejection similar to graft rejection” indeed has become “enough for us to build upon.” Immunotherapies that strive to harness our body’s immune defence against tumours have emerged as a great hope of oncology. When Harold Varmus, who had shared the Nobel Prize in Physiology or Medicine 1989 with Michael Bishop “for their discovery of the cellular origin of retroviral oncogenes” came to Lindau for the first time in 2015, he referred to these promising perspectives[21] in his lecture “From proto-oncogenes to precision oncology”.
(00:26:07 - 00:27:38)
The hope of immunotherapies
The principle of checkpoint inhibition that Varmus emphasizes, has long been successfully translated from bench to bedside. T cells have to send both stimulating and inhibiting signals to control the magnitude and duration of our immune responses. Therefore, they are equipped with certain receptors that serve as negative checkpoints to prevent our immune system from overshooting reactions. In 1996, James Allison and his group demonstrated that the blockade of the checkpoint receptor CTLA-4 by a specific antibody named ipilimumab results in the rejection of solid tumor grafts. Further investigation into this mechanism revealed additional modes of action of this antibody. This lead to its development for the treatment of melanoma. The clinical trials with the drug showed impressive results in overall survival rates of patients. In 2011, ipilimumab was the first checkpoint inhibitor to be approved by the FDA.
An application of engineered T cells has in the meantime also become a clinical reality in the treatment of certain advanced forms of leukaemia. It was invented by Carl June and his group and is in fact an individualised combined gene and immune therapy. It is based on the principle to take cells from the blood of a patient and extracorporeally equip them with a chimeric antigen receptor (CAR), namely, an antibody targeted to a specific tumour antigen. The first CAR-T cell therapy under the name of Tisagenlecleucel has been approved by the FDA in 2017.
Another potential immunotherapy, which has not yet reached the market, is based on messenger RNA molecules that carry the individual genetic information of a patient’s tumour. This personal tumour pattern needs first to be identified by gene sequencing and bioinformatic analysis. Subsequently, via their mRNA templates, selected individual tumour proteins are presented to the patient’s dendritic cells. It is hoped that the dendritic cells in turn will activate T cells to massively attack the tumour antigens.
Immunology has come a long way since its foundation as a scientific discipline more than a dozen decades ago. Yet it looks fresh and young as if it only had just begun. Immunologists have delivered a lot of meaningful medical solutions, and they have also opened the window for many more meaningful questions. Certainly, many more Nobel Prizes will be awarded for new insights into the complexity of our immune system in the future.
Footnotes
[1] cf. Stefan Kaufmann. Immunology’s foundation: the 100-year anniversary of the Nobel prize to Paul Ehrlich and Elie Metchnikoff. Nat. Immunol. 9. 705-711 (2008).
[2] Stefan Kaufmann. Immunology’s foundation, l.c.
[3] https://www.nobelprize.org/nobel_prizes/medicine/laureates/1908/press.html
[4] All quotes from Mechnikov’s Nobel Lecture: https://www.nobelprize.org/nobel_prizes/medicine/laureates/1908/mechnikov-lecture.html
[5] Original German formulation: “Die Immunität von Kaninchen und Mäusen, die gegen Tetanus immunisiert sind, beruht auf der Fähigkeit der zellenfreien Blutflüssigkeit, die toxischen Substanzen, welche die Tetanusbazillen produzieren, unschädlich zu machen.” cf. Behring/ Kitasato. Ueber das Zustandekommen der Diphterie-Immunität und der Tetanus-Immunität bei Tieren. Deutsche Medizinische Wochenschrift. 49/1890, https://doi.org/10.17192/eb2013.0164
[6] It has to be taken into consideration that the first passive immunization had been conducted by Edward Jenner already in 1796, as the Karolinska Institute acknowledged in its press release on occasion of the NP in Physiology or Medicine 1908: “It was, therefore, rightly considered to be an epoch-making and blessed moment in the history of medicine when Edward Jenner introduced, more than a hundred years ago, protective vaccination with cow-pox substance which can give immunity against a disease, namely smallpox, the ravages of which the present generation can hardly imagine.” https://www.nobelprize.org/nobel_prizes/medicine/laureates/1908/press.html
[7] cf. Stefan Kaufmann. Emil von Behring: translational medicine at the dawn of immunology. Nat. Rev. Immunol. 17, 341-343 (2017)
[8] https://www.nobelprize.org/nobel_prizes/medicine/laureates/1908/ehrlich-lecture.pdf
[9] Arthur M. Silverstein. A History of Immunology. Second Edition 2009, p. 456
[10] ibid., p. 457
[11] Niels Jerne quoted by Hodgkin/Heath/Baxter: The clonal selection theory: 50 years since the revolution. Nat. Immunol. 8, 1019-1026 (2007), reprinted in: Nature Milestones Antibodies, December 2016, p. S40-S44
[12] F. M. Burnet. A Modification of Jerne’s theory of antibody production using the concept of clonal selection. The Australian Journal of Science. Vol. 20, Nr. 3, 21 October 1957
[13] Hodgkin/Heath/Baxter, l.c.
[14] Besides on information gained from the quoted Lindau lectures, the author also relied on two textbooks, namely L. Rind, A. Kruse, H. Haase. Immunologie für Einsteiger. 2nd edition, 2015; Bruce Alberts et al. Molecular Biology of the Cell, 6th edition, 2015, Chapter 23 and 24.
[15] Molecular Biology of the Cell, l.c., p. 1264
[16] Immunologie für Einsteiger, l.c., p. 34
[17] https://www.nobelprize.org/nobel_prizes/medicine/laureates/1984/press.html
[18] Immunologie für Einsteiger, l.c., p. 253
[19] Köhler, Milstein. Continous cultures of fused cells secreting antibody of predefined specificity. Nature 256, 495-497 (1975), quoted by Nature Milestones, l.c.
[20] Nature Milestones, l.c., p. S2
[21] An interesting blog on the prospects of immunotherapy was posted by Melissae Fallet on the website of the Lindau Nobel Laureate Meetings in October 2017, cf. http://www.lindau-nobel.org/blog-immunotherapy-the-next-revolution-in-cancer-treatment/