Prof. Dr. Steven Weinberg > Research Profile
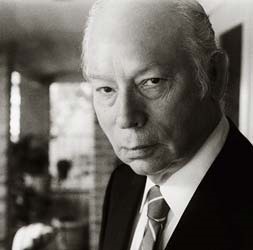
by Roberto Lalli
Steven Weinberg Nobel Prize in Physics 1979 together with Sheldon Glashow and Abdus Salam
"for their contributions to the theory of the unified weak and electromagnetic interaction between elementary particles, including, inter alia, the prediction of the weak neutral current".
Steven Weinberg is an American theoretical physicist, who currently holds the Josey Regental Chair in Science at the University of Texas at Austin. He is one of the founding fathers of the Standard Model of particle physics, and is commonly regarded as being among the most influential living theoreticians. The theory of interactions between leptons, which Weinberg developed in 1967 – along with the similar one developed independently by Abdus Salam - is currently considered as the standard description of the unification of electromagnetic and weak interactions. After further theoretical developments and experimental confirmation, the electroweak theory gained Weinberg the 1979 Nobel Prize in Physics, which he shared with Salam and Sheldon L. Glashow, the latter having anticipated the same theoretical scheme in 1961. Weinberg’s copious production of papers and books made his impact on physics go well beyond the formulation of the Standard Model electroweak theory. In his research papers, Weinberg has been working on the theoretical development of different topics, including the unification of forces, cosmology, astrophysics, supersymmetry, and supergravity. His research has been often driven by a strong commitment towards the search for what he calls the “final theory.” Not only did he produce original research in this context, he has also assumed a leading role within the community of physicists. On various occasions, he has drawn the lines that laid the fundamental methodological and mathematical principles, which serves as constraints for the construction of such a unifying theory. Moreover, he presented his views in textbooks as well as books for general public. By means of these books, Weinberg has been playing a strong role in several cultural debates concerning the aim of science, its methodology and its relationship with religion.
Early Works in the Renormalization of Quantum Field Theory
Weinberg was born in New York City on May 3, 1933. Very early in his life, Weinberg was attracted to science and was encouraged to follow his interests by his parents. When he was 16 years old, he already focused on theoretical physics as the core of his intellectual curiosity. He enrolled at the Bronx High School of Science – a public school focused on the teaching of sciences, which had been recently founded. One of his classmates was Glashow, with whom he would share the Nobel Prize some thirty years later. Soon after having received their diploma in 1950, both Weinberg and Glashow began their bachelor’s studies at Cornell University, where Weinberg graduated in 1954. Soon after, he spent a year of graduate studies at the Institute of Theoretical Physics in Copenhagen (now the Niels Bohr Institute), and completed his PhD at Princeton University in 1957. Weinberg’s supervisor was the American theoretical physicist Sam Bard Treiman who was developing a deep interest in weak interactions just in that period. In his dissertation, Weinberg addressed issues concerning the renormalization of quantum field theories of strong and weak interactions. It is noteworthy that this approach would be crucial in Weinberg’s elaboration of the electroweak theory ten years later.
The period in which Weinberg was completing his dissertation was characterised by considerable theoretical turmoil in particle physics. Between 1956 and 1957, the experimental discovery of parity violation in weak interactions was prompting physicists to reconfigure their theoretical descriptions of these processes. At the same time, some theorists were trying to build a classification of strong interacting particles in order to make sense of the numerous particles that were being discovered in cosmic ray observations and particle accelerators. Moreover, by the early 1950s many theorists had lost confidence in the venture of constructing a reliable quantum field theory of elementary particle interactions. It was most common to direct theoretical efforts towards the creation of phenomenological models. Until the 1970s, quantum electrodynamics (QED) was the only quantum field theory that was broadly accepted.
In the 1940s, physicists were more optimistic about the possibility to formulate a realistic quantum field theory of other particle interactions. The renormalization techniques developed by S.-I. Tomonaga, J. Schwinger, R. Feynman – who all shared the 1965 Nobel Prize in Physics - and F. Dyson had proved very useful in solving the divergence problems affecting QED. Infinities were cancelled out by means of a redefinition of the parameters such as mass and charge. These procedures - and, in particular, the Feynman diagrams - were soon favourably received because calculations made in renormalized QED led to accurate agreements with experimental discoveries such as the Lamb effect and the anomalous magnetic momentum of the electron.
While in the late 1940s these successes had revitalised the research for a quantum field theoretical description of the other particle interactions, by the early 1950s physicists gave up the hope that such models might also correctly describe weak and strong interactions. Renormalization was understood as a necessary constraint for a quantum field theory to be acceptable, and only few field theories could be made renormalizable. It was necessary, e.g., that the coupling constants related to the interactions had certain dimensions. The Fermi coupling constant of the weak interactions did not fit these requirements, and, consequently, a quantum field theory of weak interaction was considered as necessarily unrenormalizable. As for the strong interactions, the renormalization did not allow for useful calculations because the coupling constant was too large. It was not possible to build a hierarchy among the possible interactions between the particle fields. For this reason, many believed that no relationship with experimental data could be deduced through a renormalized quantum field theory of strong interactions.
In his Nobel lecture, Weinberg emphasised that he welcomed the renormalization theory because “limitations of this sort are, after all, what we most want, not mathematical methods which can make sense of an infinite variety of physically irrelevant theories, but methods which carry constraints, because these constraints may point the way toward the one true theory.” During his postdoctoral period at Columbia University and his years as a faculty member of the Berkeley University, Weinberg continued to work on quantum field theory and particle physics. Influenced by Dyson’s “lucid papers,” Weinberg developed a thorough knowledge of Feynman’s renormalization rules and - in 1959 - provided rigorous proof that ultraviolet divergences cancel out to all orders of perturbation theory of quantum electrodynamics by means of a mechanism that is sometimes called Weinberg’s convergence theorem. While renormalization theory continued to hold a strong methodological place in Weinberg’s research, around 1960 Weinberg began to be captivated by a different concept that was being transferred from solid-state physics to particle physics: the spontaneous symmetry breaking of an underlying symmetry of the physical laws.
The Unification of Forces and the Spontaneous Symmetry Breaking
In 1954, the Chinese-American theoretical physicist C. N. Yang – who would share the 1957 Nobel Prize in Physics with T. D. Lee for their work on parity violation – and the American PhD student R. Mills had developed a generalisation of gauge field theories. Starting from the gauge invariance of electrodynamics described by the U(1) symmetry, Yang and Mills put forward a general equation invariant with respect to SU(N) transformations – transformations governed by unitary N-dimensional matrices with determinant 1. Contrary to what happens in the electromagnetic case, the equations of the
b
fields – needed in order to make the equation of the Yang-Mills theory SU(N) invariant - were nonlinear; namely, they were part of their own source. This led to several complications, but many looked at this theory as a possible framework for elaborating a quantum field theory of the strong interactions.
Around 1957, some theorists - including Schwinger and Glashow - began considering the possibility that the Yang-Mills theory might allow for a unification of electromagnetic and weak interactions in the same mathematical framework. The rationale underlying this idea was that the electromagnetic and the weak interactions presented similar features: Both interactions were thought to be universal and their coupling constants seemed to have a similar value. In addition, a quantum field theory of weak interactions alone could not be made renormalizable.
This unification programme met several difficulties, however. While the quantum of the electromagnetic field is massless, the short range of weak interactions implied that the hypothesised vector bosons of weak interactions were very heavy. A second problem emphasised by Glashow was that electromagnetic interactions do conserve parity, while weak interactions do not. In 1961, Glashow devised a promising scheme that overcomes the latter difficulty by proposing that the symmetry gauge was SU(2)xU(1). A consequence of this approach was that there were four gauge bosons: three heavy bosons mediating weak forces – in current terminology the two electrically charged Ws and the electrically neutral Z - and one massless gauge boson carrying electromagnetic force – the photon γ. While Glashow’s theory solved the parity anomaly problem, the explanation it provided for the mass differences between the weak bosons and the photon was not convincing.
One of the reasons why Glashow’s theory was not developed immediately was the slow evolution of the theoretical understanding of the Yang-Mills non-Abelian theory. Soon after the theory was proposed in 1954, many suspected that the gauge bosons - the quanta of the compensating vector field
b
- should be massless in order to not destroy gauge invariance. But no such particles had ever been observed. Following progress made in solid-state physics, some theorists - including the 2008 Nobel Prize Laureate in physics Y. Nambu and his co-worker G. Jona-Lasinio - proposed that the gauge bosons might acquire mass from some sort of spontaneous breaking of the continuous local gauge symmetry. Soon spontaneous symmetry breaking became an important research stream in particle physics. Weinberg himself was deeply fascinated by the subject and tried to apply this concept to the behaviours of strong-interacting particles.
These attempts, however, were hindered by the formulation of the Goldstone theory. In the same year that Glashow published his electroweak theory, J. Goldstone provided a model in which the vacuum spontaneously broke the symmetry while the equations of motion retained it. In the Goldstone – also called Goldstone-Nambu - model, the spontaneous symmetry breaking of a continuous symmetry - such as the gauge symmetry was - necessarily implied the existence of massless bosons - soon christened Goldstone bosons. In 1963, Weinberg made a major contribution to this development by collaborating with Salam and Goldstone on the generalisation of this proof in the context of quantum field theory. The Goldstone-Salam-Weinberg demonstration seemed to imply that quantum field theories of elementary interactions were definitely ruled out by the fact that the Goldstone boson has never been observed.
Many theoreticians - including the 2013 Nobel Laureates in Physics P. Higgs and F. Englert - tried to overcome this difficulty by elaborating a mechanism through which the gauge bosons acquire mass because of the spontaneous breaking of the continuous symmetry, while “eating” the Goldstone bosons. This mechanism - now called the Higgs phenomenon - provided a fundamental step towards the creation of the Standard Model unified electroweak theory. Weinberg soon became acquainted with this mechanism and explored its implications for the strong interactions and the SU(3) Yang-Mills theory. Through this somewhat indirect route, Weinberg conceived of the idea that the Higgs phenomenon might be employed for the unified description of electromagnetic and weak interactions. In 1967 - when he was a visiting professor at the Massachusetts Institute of Technology - Weinberg published the 3-page paper “A theory of leptons.” Here, he argued that Glashow’s SU(2)xU(1) gauge symmetry was indeed the right scheme for the electroweak interactions provided that the W and Z gauge bosons acquired their masses through the Higgs mechanism. A few months later, Salam proposed an essentially equivalent model.
By 1968, the Standard Model electroweak theory, that is the Glashow-Salam-Weinberg SU(2)xU(1) theory, was born. But several experimental and theoretical developments were necessary before the theory came to be accepted in the following decade.
From an Unfavourable Reception to the Acceptance of the Standard Model
Although Weinberg and Salam solved some of the most disturbing problems affecting Glashow’s theory, most physicists paid no attention to their model in the following years. Weinberg himself never quoted his theory until 1971. The main problem was that renormalization was considered to be an essential feature for a quantum field theory to gain respectability, and there was no proof that the Weinberg-Salam model was renormalizable. Weinberg strongly believed in the importance of this principle in the choice among competing theories, and he assumed that his electroweak theory was renormalizable. However, a formal proof was missing. This problem was solved only in 1971 when the Dutch PhD candidate G. ‘tHooft developed a renormalization methodology first put forward by his advisor M. Veltman, which demonstrated that Yang-Mills theories whose gauge bosons acquire mass through the Higgs mechanism are renormalizable. Weinberg later recalled that he was unsure as to whether ‘tHooft’s demonstration really demonstrated that the SU(2)xU(1) electroweak theory was renormalizable. Further theoretical elaborations immediately convinced him that the theory he and Salman had developed was indeed renormalizable, and then a good candidate for the description of elementary interactions.
A second problem affected the reception of their theory, though. It was clear soon after Glashow first proposed the SU(2)xU(1) scheme that the theory required the existence of weak neutral currents related to the exchange of the gauge boson Z. After Weinberg became convinced of the validity of the theory, he began working out several implications of the theory as well as its relationship with the strong interactions. Weinberg’s calculations drove the experimental research that was being done in various facilities. Several technical improvements were made in order to test whether the neutral currents really existed and convincing empirical confirmation began to appear already in 1973. In 1978, the observation of parity-violating neutral currents involving charged leptons persuaded many physicist of the validity of the Glashow-Salam-Weinberg theory. Following this empirical confirmation, the three theoretical physicists were jointly awarded the 1979 Nobel Prize “for their contributions to the theory of the unified weak and electromagnetic interaction between elementary particles, including, inter alia, the prediction of the weak neutral current.”
However, the essential objects of the theories - the heavy gauge bosons - had not been observed yet. It took other four years for experimenters to produce significant evidence of the existence of these particles. The belief in the validity of the model was so widespread that two of the main researchers involved in these experimental findings - C. Rubbia and S. van der Meer - were awarded the Nobel Prize in Physics the very same year after the announcement of the discovery of the field particles W and Z. In 1984, the electroweak theory and the simultaneously developed chromodynamics of strong interactions had reached the status of the standard model for the description of the particle interactions – and so they have been called ever since.
Cultural Debates
While continuing to develop the Standard Model electroweak theory and its implications, Weinberg made valuable research in other fields and opened entirely new research streams. Among his main contributions, one recalls his works on chromodynamics in the early 1970s and the definition of quark confinement, Weinberg’s attempts to produce quantum field theoretical models that extend the Standard Model with his works on supersymmetry (usually called SUSY) and the supersymmetrical theory of gravity called supergravity. He also published two important textbooks that strongly influenced younger generations of physicists:
Gravitation and Cosmology
published in 1972 and
Quantum Theory of Fields
, whose three volumes were published between 1995 and 2000.
Apart from his work in theoretical physics, Weinberg was actively involved in various debates concerning the foundations of physics as well as the role and methodology of science. He has been trying to push his views and to popularise them through different means, including articles for the
New York Review of Books
. Weinberg took a strong position in the emergence-reductionism debate, which sparked strong controversies among physicists of different disciplines on what counts as a fundamental research. He was an outspoken supporter of the reductionist perspective by arguing for a sort of hierarchy between research fields in science, and more specifically in theoretical physics. In this context, he testified before the U.S. Congress to provide arguments in favour of the construction of the Superconducting Super Collider (SSC) – a high-energy particle accelerator whose construction began in the late 1980s. Weinberg became deeply embittered when the project was eventually discarded in 1993 after about two billion dollars had already been spent. In the 1990s, he also publicly criticised those social scientists that were promoting an anti-realist and constructionist view of science in the series of intellectual controversies that were christened the “science wars.” His strong commitments to these and other cultural debates have made him one of the most influential contemporary theoretical physicists in the public sphere. They show, moreover, that Weinberg has never conceived the role of a theoretical physicist to be that of a solitary researcher far from the needs of the society.
Bibliography
Fitch V. L., & Rosner L. (1995) Elementary Particle Physics in the Second Half of the Twentieth Century. In Brown, L., Pippard, B., & Pais, A. (Eds.). Twentieth Century Physics (Vol. 2). AIP, New York, pp. 635-794.
Hoddeson, L., Brown, L. M., Riordan, M. & Dresden, M. (1995) The Rise of the Standard Model: Particle Physics in the 1960s and the 1970s. Cambridge University Press, Cambridge.
Pais, A. (1986) Inward Bound: Of Matter and Forces in the Physical World. Clarendon Press, Oxford.
Weinberg, S. (1967) A theory of leptons. Phys. Rev. Lett. 19: 515-524.
Weinberg, S. (1977) The search for unity: Notes for a history of quantum field theory. Daedalus 106: 17-35.
Weinberg, S. (1980) Conceptual foundations of the unified theory of weak and electromagnetic interactions. Reviews of Modern Physics 52: 515-524.
Weinberg, S. (1993) Dreams of a final theory: The search for the fudamental laws of nature. Vintage, London.
Weinberg, S. (1996) The quantum theory of fields (Vol. 1): Foundations. Cambridge University Press, Cambridge.
Steven Weinberg Nobel Prize in Physics 1979 together with Sheldon Glashow and Abdus Salam
"for their contributions to the theory of the unified weak and electromagnetic interaction between elementary particles, including, inter alia, the prediction of the weak neutral current".
Steven Weinberg is an American theoretical physicist, who currently holds the Josey Regental Chair in Science at the University of Texas at Austin. He is one of the founding fathers of the Standard Model of particle physics, and is commonly regarded as being among the most influential living theoreticians. The theory of interactions between leptons, which Weinberg developed in 1967 – along with the similar one developed independently by Abdus Salam - is currently considered as the standard description of the unification of electromagnetic and weak interactions. After further theoretical developments and experimental confirmation, the electroweak theory gained Weinberg the 1979 Nobel Prize in Physics, which he shared with Salam and Sheldon L. Glashow, the latter having anticipated the same theoretical scheme in 1961. Weinberg’s copious production of papers and books made his impact on physics go well beyond the formulation of the Standard Model electroweak theory. In his research papers, Weinberg has been working on the theoretical development of different topics, including the unification of forces, cosmology, astrophysics, supersymmetry, and supergravity. His research has been often driven by a strong commitment towards the search for what he calls the “final theory.” Not only did he produce original research in this context, he has also assumed a leading role within the community of physicists. On various occasions, he has drawn the lines that laid the fundamental methodological and mathematical principles, which serves as constraints for the construction of such a unifying theory. Moreover, he presented his views in textbooks as well as books for general public. By means of these books, Weinberg has been playing a strong role in several cultural debates concerning the aim of science, its methodology and its relationship with religion.
Early Works in the Renormalization of Quantum Field Theory
Weinberg was born in New York City on May 3, 1933. Very early in his life, Weinberg was attracted to science and was encouraged to follow his interests by his parents. When he was 16 years old, he already focused on theoretical physics as the core of his intellectual curiosity. He enrolled at the Bronx High School of Science – a public school focused on the teaching of sciences, which had been recently founded. One of his classmates was Glashow, with whom he would share the Nobel Prize some thirty years later. Soon after having received their diploma in 1950, both Weinberg and Glashow began their bachelor’s studies at Cornell University, where Weinberg graduated in 1954. Soon after, he spent a year of graduate studies at the Institute of Theoretical Physics in Copenhagen (now the Niels Bohr Institute), and completed his PhD at Princeton University in 1957. Weinberg’s supervisor was the American theoretical physicist Sam Bard Treiman who was developing a deep interest in weak interactions just in that period. In his dissertation, Weinberg addressed issues concerning the renormalization of quantum field theories of strong and weak interactions. It is noteworthy that this approach would be crucial in Weinberg’s elaboration of the electroweak theory ten years later.
The period in which Weinberg was completing his dissertation was characterised by considerable theoretical turmoil in particle physics. Between 1956 and 1957, the experimental discovery of parity violation in weak interactions was prompting physicists to reconfigure their theoretical descriptions of these processes. At the same time, some theorists were trying to build a classification of strong interacting particles in order to make sense of the numerous particles that were being discovered in cosmic ray observations and particle accelerators. Moreover, by the early 1950s many theorists had lost confidence in the venture of constructing a reliable quantum field theory of elementary particle interactions. It was most common to direct theoretical efforts towards the creation of phenomenological models. Until the 1970s, quantum electrodynamics (QED) was the only quantum field theory that was broadly accepted.
In the 1940s, physicists were more optimistic about the possibility to formulate a realistic quantum field theory of other particle interactions. The renormalization techniques developed by S.-I. Tomonaga, J. Schwinger, R. Feynman – who all shared the 1965 Nobel Prize in Physics - and F. Dyson had proved very useful in solving the divergence problems affecting QED. Infinities were cancelled out by means of a redefinition of the parameters such as mass and charge. These procedures - and, in particular, the Feynman diagrams - were soon favourably received because calculations made in renormalized QED led to accurate agreements with experimental discoveries such as the Lamb effect and the anomalous magnetic momentum of the electron.
While in the late 1940s these successes had revitalised the research for a quantum field theoretical description of the other particle interactions, by the early 1950s physicists gave up the hope that such models might also correctly describe weak and strong interactions. Renormalization was understood as a necessary constraint for a quantum field theory to be acceptable, and only few field theories could be made renormalizable. It was necessary, e.g., that the coupling constants related to the interactions had certain dimensions. The Fermi coupling constant of the weak interactions did not fit these requirements, and, consequently, a quantum field theory of weak interaction was considered as necessarily unrenormalizable. As for the strong interactions, the renormalization did not allow for useful calculations because the coupling constant was too large. It was not possible to build a hierarchy among the possible interactions between the particle fields. For this reason, many believed that no relationship with experimental data could be deduced through a renormalized quantum field theory of strong interactions.
In his Nobel lecture, Weinberg emphasised that he welcomed the renormalization theory because “limitations of this sort are, after all, what we most want, not mathematical methods which can make sense of an infinite variety of physically irrelevant theories, but methods which carry constraints, because these constraints may point the way toward the one true theory.” During his postdoctoral period at Columbia University and his years as a faculty member of the Berkeley University, Weinberg continued to work on quantum field theory and particle physics. Influenced by Dyson’s “lucid papers,” Weinberg developed a thorough knowledge of Feynman’s renormalization rules and - in 1959 - provided rigorous proof that ultraviolet divergences cancel out to all orders of perturbation theory of quantum electrodynamics by means of a mechanism that is sometimes called Weinberg’s convergence theorem. While renormalization theory continued to hold a strong methodological place in Weinberg’s research, around 1960 Weinberg began to be captivated by a different concept that was being transferred from solid-state physics to particle physics: the spontaneous symmetry breaking of an underlying symmetry of the physical laws.
The Unification of Forces and the Spontaneous Symmetry Breaking
In 1954, the Chinese-American theoretical physicist C. N. Yang – who would share the 1957 Nobel Prize in Physics with T. D. Lee for their work on parity violation – and the American PhD student R. Mills had developed a generalisation of gauge field theories. Starting from the gauge invariance of electrodynamics described by the U(1) symmetry, Yang and Mills put forward a general equation invariant with respect to SU(N) transformations – transformations governed by unitary N-dimensional matrices with determinant 1. Contrary to what happens in the electromagnetic case, the equations of the
b
fields – needed in order to make the equation of the Yang-Mills theory SU(N) invariant - were nonlinear; namely, they were part of their own source. This led to several complications, but many looked at this theory as a possible framework for elaborating a quantum field theory of the strong interactions.
Around 1957, some theorists - including Schwinger and Glashow - began considering the possibility that the Yang-Mills theory might allow for a unification of electromagnetic and weak interactions in the same mathematical framework. The rationale underlying this idea was that the electromagnetic and the weak interactions presented similar features: Both interactions were thought to be universal and their coupling constants seemed to have a similar value. In addition, a quantum field theory of weak interactions alone could not be made renormalizable.
This unification programme met several difficulties, however. While the quantum of the electromagnetic field is massless, the short range of weak interactions implied that the hypothesised vector bosons of weak interactions were very heavy. A second problem emphasised by Glashow was that electromagnetic interactions do conserve parity, while weak interactions do not. In 1961, Glashow devised a promising scheme that overcomes the latter difficulty by proposing that the symmetry gauge was SU(2)xU(1). A consequence of this approach was that there were four gauge bosons: three heavy bosons mediating weak forces – in current terminology the two electrically charged Ws and the electrically neutral Z - and one massless gauge boson carrying electromagnetic force – the photon γ. While Glashow’s theory solved the parity anomaly problem, the explanation it provided for the mass differences between the weak bosons and the photon was not convincing.
One of the reasons why Glashow’s theory was not developed immediately was the slow evolution of the theoretical understanding of the Yang-Mills non-Abelian theory. Soon after the theory was proposed in 1954, many suspected that the gauge bosons - the quanta of the compensating vector field
b
- should be massless in order to not destroy gauge invariance. But no such particles had ever been observed. Following progress made in solid-state physics, some theorists - including the 2008 Nobel Prize Laureate in physics Y. Nambu and his co-worker G. Jona-Lasinio - proposed that the gauge bosons might acquire mass from some sort of spontaneous breaking of the continuous local gauge symmetry. Soon spontaneous symmetry breaking became an important research stream in particle physics. Weinberg himself was deeply fascinated by the subject and tried to apply this concept to the behaviours of strong-interacting particles.
These attempts, however, were hindered by the formulation of the Goldstone theory. In the same year that Glashow published his electroweak theory, J. Goldstone provided a model in which the vacuum spontaneously broke the symmetry while the equations of motion retained it. In the Goldstone – also called Goldstone-Nambu - model, the spontaneous symmetry breaking of a continuous symmetry - such as the gauge symmetry was - necessarily implied the existence of massless bosons - soon christened Goldstone bosons. In 1963, Weinberg made a major contribution to this development by collaborating with Salam and Goldstone on the generalisation of this proof in the context of quantum field theory. The Goldstone-Salam-Weinberg demonstration seemed to imply that quantum field theories of elementary interactions were definitely ruled out by the fact that the Goldstone boson has never been observed.
Many theoreticians - including the 2013 Nobel Laureates in Physics P. Higgs and F. Englert - tried to overcome this difficulty by elaborating a mechanism through which the gauge bosons acquire mass because of the spontaneous breaking of the continuous symmetry, while “eating” the Goldstone bosons. This mechanism - now called the Higgs phenomenon - provided a fundamental step towards the creation of the Standard Model unified electroweak theory. Weinberg soon became acquainted with this mechanism and explored its implications for the strong interactions and the SU(3) Yang-Mills theory. Through this somewhat indirect route, Weinberg conceived of the idea that the Higgs phenomenon might be employed for the unified description of electromagnetic and weak interactions. In 1967 - when he was a visiting professor at the Massachusetts Institute of Technology - Weinberg published the 3-page paper “A theory of leptons.” Here, he argued that Glashow’s SU(2)xU(1) gauge symmetry was indeed the right scheme for the electroweak interactions provided that the W and Z gauge bosons acquired their masses through the Higgs mechanism. A few months later, Salam proposed an essentially equivalent model.
By 1968, the Standard Model electroweak theory, that is the Glashow-Salam-Weinberg SU(2)xU(1) theory, was born. But several experimental and theoretical developments were necessary before the theory came to be accepted in the following decade.
From an Unfavourable Reception to the Acceptance of the Standard Model
Although Weinberg and Salam solved some of the most disturbing problems affecting Glashow’s theory, most physicists paid no attention to their model in the following years. Weinberg himself never quoted his theory until 1971. The main problem was that renormalization was considered to be an essential feature for a quantum field theory to gain respectability, and there was no proof that the Weinberg-Salam model was renormalizable. Weinberg strongly believed in the importance of this principle in the choice among competing theories, and he assumed that his electroweak theory was renormalizable. However, a formal proof was missing. This problem was solved only in 1971 when the Dutch PhD candidate G. ‘tHooft developed a renormalization methodology first put forward by his advisor M. Veltman, which demonstrated that Yang-Mills theories whose gauge bosons acquire mass through the Higgs mechanism are renormalizable. Weinberg later recalled that he was unsure as to whether ‘tHooft’s demonstration really demonstrated that the SU(2)xU(1) electroweak theory was renormalizable. Further theoretical elaborations immediately convinced him that the theory he and Salman had developed was indeed renormalizable, and then a good candidate for the description of elementary interactions.
A second problem affected the reception of their theory, though. It was clear soon after Glashow first proposed the SU(2)xU(1) scheme that the theory required the existence of weak neutral currents related to the exchange of the gauge boson Z. After Weinberg became convinced of the validity of the theory, he began working out several implications of the theory as well as its relationship with the strong interactions. Weinberg’s calculations drove the experimental research that was being done in various facilities. Several technical improvements were made in order to test whether the neutral currents really existed and convincing empirical confirmation began to appear already in 1973. In 1978, the observation of parity-violating neutral currents involving charged leptons persuaded many physicist of the validity of the Glashow-Salam-Weinberg theory. Following this empirical confirmation, the three theoretical physicists were jointly awarded the 1979 Nobel Prize “for their contributions to the theory of the unified weak and electromagnetic interaction between elementary particles, including, inter alia, the prediction of the weak neutral current.”
However, the essential objects of the theories - the heavy gauge bosons - had not been observed yet. It took other four years for experimenters to produce significant evidence of the existence of these particles. The belief in the validity of the model was so widespread that two of the main researchers involved in these experimental findings - C. Rubbia and S. van der Meer - were awarded the Nobel Prize in Physics the very same year after the announcement of the discovery of the field particles W and Z. In 1984, the electroweak theory and the simultaneously developed chromodynamics of strong interactions had reached the status of the standard model for the description of the particle interactions – and so they have been called ever since.
Cultural Debates
While continuing to develop the Standard Model electroweak theory and its implications, Weinberg made valuable research in other fields and opened entirely new research streams. Among his main contributions, one recalls his works on chromodynamics in the early 1970s and the definition of quark confinement, Weinberg’s attempts to produce quantum field theoretical models that extend the Standard Model with his works on supersymmetry (usually called SUSY) and the supersymmetrical theory of gravity called supergravity. He also published two important textbooks that strongly influenced younger generations of physicists:
Gravitation and Cosmology
published in 1972 and
Quantum Theory of Fields
, whose three volumes were published between 1995 and 2000.
Apart from his work in theoretical physics, Weinberg was actively involved in various debates concerning the foundations of physics as well as the role and methodology of science. He has been trying to push his views and to popularise them through different means, including articles for the
New York Review of Books
. Weinberg took a strong position in the emergence-reductionism debate, which sparked strong controversies among physicists of different disciplines on what counts as a fundamental research. He was an outspoken supporter of the reductionist perspective by arguing for a sort of hierarchy between research fields in science, and more specifically in theoretical physics. In this context, he testified before the U.S. Congress to provide arguments in favour of the construction of the Superconducting Super Collider (SSC) – a high-energy particle accelerator whose construction began in the late 1980s. Weinberg became deeply embittered when the project was eventually discarded in 1993 after about two billion dollars had already been spent. In the 1990s, he also publicly criticised those social scientists that were promoting an anti-realist and constructionist view of science in the series of intellectual controversies that were christened the “science wars.” His strong commitments to these and other cultural debates have made him one of the most influential contemporary theoretical physicists in the public sphere. They show, moreover, that Weinberg has never conceived the role of a theoretical physicist to be that of a solitary researcher far from the needs of the society.
Bibliography
Fitch V. L., & Rosner L. (1995) Elementary Particle Physics in the Second Half of the Twentieth Century. In Brown, L., Pippard, B., & Pais, A. (Eds.). Twentieth Century Physics (Vol. 2). AIP, New York, pp. 635-794.
Hoddeson, L., Brown, L. M., Riordan, M. & Dresden, M. (1995) The Rise of the Standard Model: Particle Physics in the 1960s and the 1970s. Cambridge University Press, Cambridge.
Pais, A. (1986) Inward Bound: Of Matter and Forces in the Physical World. Clarendon Press, Oxford.
Weinberg, S. (1967) A theory of leptons. Phys. Rev. Lett. 19: 515-524.
Weinberg, S. (1977) The search for unity: Notes for a history of quantum field theory. Daedalus 106: 17-35.
Weinberg, S. (1980) Conceptual foundations of the unified theory of weak and electromagnetic interactions. Reviews of Modern Physics 52: 515-524.
Weinberg, S. (1993) Dreams of a final theory: The search for the fudamental laws of nature. Vintage, London.
Weinberg, S. (1996) The quantum theory of fields (Vol. 1): Foundations. Cambridge University Press, Cambridge.