Prof. Dr. Ernest Thomas Sinton Walton > Research Profile
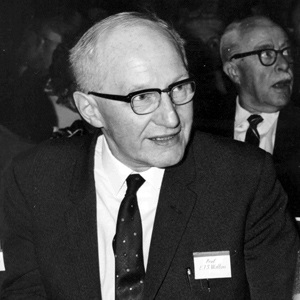
by Luisa Bonolis
Ernest Thomas Sinton Walton
Nobel Prize in Physics 1951 together with John Cockcroft
"for their pioneer work on the transmutation of atomic nuclei by artificially accelerated atomic particles".
From Dublin to the Nursery of the Cavendish Laboratory
Ernest Walton was born on October 6, 1903, in Dungarvan, on the south coast of Ireland. His aptitude in mathematics and science was evident at a very early age. He had a gift for making things, too, and liked to be given tools to equip what grew into a sophisticated home workshop. He continued his studies at the Methodist College in Belfast, where he proved a star pupil, and did brilliant work in mathematics and physics. In 1922 he was awarded a scholarship and entered Trinity College, Dublin, where he graduated with highest honours in mathematics and experimental physics in 1926. He stayed on as a postgraduate, choosing physics because it allowed him to use his hands as well as his brain. His research supervisor set him the task of studying the flow of liquids past cylinders, a matter of importance in civil engineering and aeronautics. Using an elegant apparatus of his own creation he photographed the vortices created in the liquid at each stage and then described the rules governing their formation and behaviour. After receiving his M.S. degree in 1927 he succeeded in being awarded a research scholarship from the Royal Commission for the Exhibition of 1851, and thus went to Cambridge University to work at the Cavendish Laboratory under Ernest Rutherford.
Walton arrived at Cambridge in fall of 1927 and joined what was a remarkable community. Rutherford and Chadwick directed the work of the Cavendish towards the study of the nucleus, widely viewed as the most exciting field in all science. All young researchers were required to spend at least a little time in what was called the Nursery, an attic room full of discarded or temporarily unwanted apparatus, mostly junk, used as a breaking-in ground. There Walton learned the techniques used in the laboratory and passed some weeks building and testing standard apparatus, mastering vacuum equipment, gaining experience with radioactive material.
According to a strict rule Rutherford himself had established, at six o’clock each evening the Laboratory had to be closed: “If one hadn’t accomplished what one wished by six o’clock, it was unlikely that one would do so thereafter. It would be better to go home and think about what one had done today and what one was going to do tomorrow...”
Becoming an Accelerator Physicist
Research work led by Rutherford at Cavendish in those years was focused on the transmutation of the lighter elements begun by Rutherford himself at Manchester and now continuing in close collaboration with James Chadwick. It was in 1919 that Rutherford had bombarded nitrogen with alpha particles from radium, carrying out the first artificial transmutation of a nucleus, and creating “atoms of hydrogen,” which he named protons, and, as was later proved by Patrick Blackett with his cloud chamber, oxygen nuclei. This was the first observation of an artificial nuclear reaction, obtained with a natural source. From 1922 on, Chadwick had urged Rutherford to establish work in the Cavendish on the acceleration of particles with high voltages. Particles naturally emitted by radioactive sources were no longer adequate for their purposes, and during the following years scientists in several other laboratories held similar views of the need for high-speed particles and of their value as probes for studying atomic nuclei. Many nuclear physicists pinned their faith on the electrostatic route - the simplest concept of all - acceleration between the terminals of electrostatic high voltage accelerators. Work started in several laboratories in several countries to develop the electrical machines needed. This competition soon took on the aspects of a race for high voltage and for the devices needed to accelerate particles to energies sufficient to penetrate nuclei.
In December 1927, Ernst Walton completed his training in the Nursery and knocked on Rutherford’s door to discuss his research project. He had arrived with his mind filled with hydrodynamics rather than nuclear physics, but now he declared that he wanted to work on the problem of accelerating particles by electrical means. Rutherford was inclined toward the idea of electron acceleration, with the thought that this projectile, once past the orbital electrons of like charge, would be attracted to the nucleus. With its charge of +2, the alpha particle was too strongly repelled by the large number of positive charges in the nuclei of the heavier elements to cause disintegrations. To overcome this potential barrier, Rutherford thus disregarded the alpha and proton, because electrical engineers in the 1920’s could not even furnish the voltage required to match the energy of alphas from natural radioactive sources.
Following Rutherford’s idea, Walton planned to accelerate electrons around in a circular path employing an arrangement of electrical and magnetic fields that would increase their speed with every revolution. If the electrons could be maintained in the circle long enough, then in principle they might reach energies higher even than natural alpha-ray levels, although only for very short periods. There was no evidence that electrons would be capable of penetrating nuclei even at very high speeds - only alpha particles had done that - but it was worth trying. Walton began to work in the same laboratory where, since 1926, Thomas Allibone was developing a Tesla transformer for 500-600 kV to accelerate electrons. The other scientist working in the room was John Cockcroft, an electrical engineer, who had joined the Cavendish in 1922, at a time when detectors like the zinc sulphide scintillation screen and the gold-leaf electroscope, together with other very simple - even primitive - instruments, were the standard tools of the Cavendish. He too had been given a training course in the attic of the laboratory, and the difficulty he had experienced in counting in the dark the faint scintillations produced by alpha particles had made him deeply aware of the difficulty of Rutherford’s experiments. Cockcroft’s research project was related at that time to the interaction of molecules and metal surfaces, a topic very far from fundamental nuclear physics and of a more applied character.
When Walton began to work on his project at the beginning of 1928, he soon found himself in difficulty. Between January and May, he designed three different sets of apparatus, but did not find any evidence of fast-moving electrons. Towards the end of May 1928 he realised that Rutherford’s suggestions for producing fast electrons was not going to work. He was convinced of the soundness of the basic principles, even if it was clear that something was to be perfected on the technical side of the device. Such a machine was actually developed more than ten years later by Donald Kerst in US, and was called “betatron”.
Influenced by a paper published earlier by the Norwegian engineer Rolf Widerøe, Walton proposed an alternative method of accelerating positively charged particles, which became known as the “linear accelerator”. Instead of having particles circulate in a chamber, the idea was to accelerate them in a straight line by passing them along a succession of cylinders. The gaps between these cylinders would be subject to electrical fields arranged in such a way that they would cause the particles to jump forwards. Each successive jump would increase the speed of the particle. After making a few quick calculations, Rutherford agreed that the method was feasible and worth trying. Walton then began to set up an apparatus, with John Cockcroft helping to solve the engineering problems. Once again, when he tested it, he could detect no positive results.
But the whole business of accelerating particles at Cavendish was on the verge of a breakthrough, thanks to the idea of George Gamow, a young Russian theoretical physicist working at Niels Bohr’s Institute in Copenhagen.
The Tunnelling Effect, a Breakthrough for High-Voltage Particle Accelerators
In the middle of 1928, George Gamow had published a new explanation of the Geiger-Nuttal law, which related the range of an alpha particle to the half-life of the parent radioactive nucleus: the shorter the half-life, the greater the energy of the alpha particle. His explanation, based on the relatively new wave mechanics, was that the alpha particle in the nucleus escaped not by “surmounting” the energy barrier surrounding the nucleus, but by penetrating “through” it. The probability of escape depended on the energy of the particle within. Then, reflecting on why Rutherford’s alpha particles had been able to enter the nucleus of a nitrogen atom (the 1919 experiment), Gamow applied his “tunnelling” theory in an inverse manner. In a manuscript he sent to the Cavendish Laboratory in December 1928, he calculated the probability of a particle from outside penetrating into the nucleus even though its own energy was too small to surmount the barrier around the nucleus, according to ordinary classical mechanical concepts. Cockcroft saw Gamow’s manuscript and immediately realised that if Gamow was right, it would be unnecessary to accelerate particles by means of excessively large voltages to enable a nucleus to be penetrated. Between December 1928 and January 1929 he wrote a memorandum to Rutherford summarising his own calculations. Protons with an energy of only 300 000 electronvolts should be able to penetrate into a boron nucleus with a small probability, only about 6 in 1000, but this was quite acceptable, keeping in mind that even a small current of 1 microampere of positive ions provides many many millions of protons each second. In the meantime, Gamow arrived at Cavendish as a visitor and described his new work in a seminar, during which Allibone and Walton learned about the formula for the probability of nuclear penetration. Rutherford was sufficiently impressed to allow Cockcroft to go ahead and suggested that Walton, whom he considered a man of exceptional ability, who had tackled with great skill the difficult challenges related to particle acceleration, should collaborate with him in this new enterprise. This was a masterstroke, because it partnered two people with different but complementary expertise.
The Cockcroft-Walton Accelerator
It was a fortunate coincidence that, in the middle of 1931, Cockcroft and Walton had to dismantle their first preliminary apparatus, which did not produce the expected results, and move into a much larger room, with a high ceiling, which had been a lecture theatre. This provided the opportunity to start again from scratch, using an ingenious voltage multiplying circuit which Cockcroft had developed modifying one due to the German engineer M. Schenkel. The problem of the production of high velocity protons could be divided in two parts: first, the production of a stream of protons in a form suitable for acceleration, and second, the method of acceleration. In order to obtain high steady potentials, the basic idea was that of a ladder-cascade principle by which the voltage of a transformer could be rectified and multiplied several times by an arrangement of valves and condensers. They constructed a voltage quadrupling system - capable of extension to any even multiple of the transformer voltage - which used thermionic rectifiers to charge capacitors in parallel at low potential - each bearing a voltage of a few hundred volts - and discharged them in series through a load resistor to develop a high potential. The combined voltage was then connected to the accelerating column in order to accelerate protons. The rectifier system was built consisting of four straight glass cylinders placed end to end, and arranged in the form of a tower, the cylinders containing suitable electrodes and hot filaments and being evacuated continuously by a diffusion pump at earth potential. The ion source at the upper terminal consisted of a high-voltage discharge in hydrogen gas in a separate chamber with a small-diameter exit hole through which the hydrogen ions emerged into the accelerating tube. After acceleration down the column, the beam of hydrogen ions emerged through a channel in the grounded base-plate, where it was directed against the target.
In February 1932 Cockcroft and Walton were able to bring a narrow beam of 710 kilovolt protons out through a thin mica window in the base of the experimental tube, and to measure the range of the protons as a function of energy. But Rutherford was pushing for results, and on April 14 they were finally ready to bombard lithium, a light element, which would make the most suitable target. When the voltage had gone up to a reasonable value, Walton crept into the small hut under the apparatus, which protected them from the X-rays and from the high voltages. Opposite the mica window they had placed the well-tried tool of Rutherford, the fluorescent screen, a piece of paper or cardboard coated with very fine crystals of zinc sulphide, emitting small flashes of green light when struck by an alpha particle, which can be seen with a microscope. Immediately below the accelerator tube, an observation cubicle was installed, which was covered with black velvet, so that the faint scintillations by the detector might be observed. Almost at once, at an energy of 125 kilovolts, Walton saw the tiny but bright characteristic scintillations in the microscope. Rutherford arrived quickly and after some tests he said that those particles actually looked like his beloved alpha particles, which he himself had named at the beginning of the century. It appeared that the observed phenomenon could be interpreted as a lithium nucleus into which a hydrogen nucleus has penetrated breaking up into two helium nuclei, which are emitted with high energy.
After a couple of weeks a letter signed by Cockcroft and Walton appeared in Nature announcing the first major development and use of a particle accelerator. It implied some major achievements: Schrödinger’s new theory of wave mechanics and Gamow’s predictions based on it had been fully verified. For the first time an artificial nuclear reaction - the splitting of the lithium nucleus, and thus a chemical transmutation - was obtained with a substantial energy release, according to the reaction lithium-7 + proton –> helium-4 + helium-4 + energy. The mass values were furnished with great accuracy by another long-time Cavendish member, Francis Aston, who had been awarded the 1922 Nobel Prize in Chemistry “for his discovery, by means of his mass spectrograph, of isotopes, in a large number of non-radioactive elements, and for his enunciation of the whole-number rule.” The combined mass of the resulting helium nuclei is actually slightly less than the combined mass of the original lithium and hydrogen nuclei. The very small change in mass becomes energy, in good agreement with the mass balance and energy release of the observed reaction, as expected from Einstein’s mass-energy equivalence E=mc^2.
After a couple of weeks Einstein himself visited Cambridge and saw Cockcroft and Walton’s experiment writing at the time of his “astonishment and admiration” at what he had seen. Almost immediately the sensational news broke to the world. It was the first of its kind to excite the imagination of the public and to give the layperson a feeling that physicists might be working on something of world-shaking importance. The Press became very excited about the prospect of getting power from the atom, but Rutherford told them that the idea was moonshine.
The Cockcroft-Walton voltage multiplier circuit soon became a standard tool in physics laboratories and an essential part of later bigger particle accelerators, but was also used in many everyday electronic devices that require high voltages, such as X-ray machines or television sets. However, John Cockcroft and Ernest Walton had to wait until 1951 to be jointly awarded the Nobel Prize in Physics “for their pioneer work on the transmutation of atomic nuclei by artificially accelerated atomic particles.”
Bibliography
Cathcart, B. (2005) The Fly in the Cathedral. How a small group of Cambridge scientists won the race to split the atom. Pinguin Books, London
Cockcroft, (1953) J. The Rutherford Memorial Lecture. Proceedings of the Royal Society of London A 217(1128): 1-8
Devons, S. (1991) Rutherford and the science of his day. Notes and Records of the Royal Society of London 45(2): 221-242
Finch, E.C. (2007) E.T.S. Walton: Atom Splitter and Man of Peace. Dublin Historical Record 60(1): 111-123
Hartcup, G. and Allibone, T. E. (1984). Cockcroft and the Atom. Adam Hilger, Bristol
Poole, M. et al. (2007). Cockcroft’s subatomic legacy: splitting the atom, CERN Courier, December 2007. Nov 20, 2007, http://cerncourier.com/cws/article/cern/31864