Prof. Dr. Wolfgang Paul > Research Profile
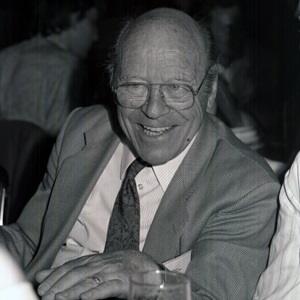
by Luisa Bonolis
Wolfgang Paul
Nobel Prize for Physics 1989 together with Norman F. Ramsey and Hans G. Dehmelt "for the development of the ion trap technique".
Becoming an Accelerator Physicist
Wolfgang Paul was born on 10 August 1913 in Lorenzkirch, a small village in Saxony, Germany. He grew up in Munich where his father was a professor of pharmaceutical chemistry at the university. His father had studied chemistry and medicine and had been a research student in Leipzig with Wilhelm Ostwald, the Nobel Laureate of 1909. So, since he was very young, Paul became familiar with the life of a scientist in a chemical laboratory and his interest in sciences was awakened quite early. After finishing the gymnasium in München he decided to become a physicist. Arnold Sommerfeld, who was a University colleague of his father, advised him to begin with an apprenticeship in precision mechanics. In the fall of1932, he began his studies at the Technische Hochschule in Munich, but after his first examination in 1934 he changed to the Technische Hochschule in Berlin where he found in Hans Kopfermann an inspiring teacher. Kopfermann, a former Ph.D. student of James Franck, had just returned from a three-year stay at the Niels Bohr Institute in Copenhagen, working in the field of hyperfine spectroscopy and nuclear moments. Both Kopferman and the theorist Richard Becker exerted a deep and lasting influence on Paul's thinking throughout the troubled years of Nazi Germany, also through their human and political attitude. All this represented the roots for his later engagement in the anti nuclear-weapon discussion and for his decision to join the group of eighteen leading nuclear researchers of the newly founded Federal Republic of Germany who opposed Chancellor Konrad Adenauer and Defence Secretary Franz-Josef Strauß's move to arm the West German army with tactical nuclear weapons. All this had important consequences in German politics.
In 1937, after his diploma exam with Hans Geiger as examinator, Paul followed his doctorate adviser Kopfermann to the University of Kiel where he had just been appointed Professor Ordinarius. For his doctoral thesis, Paul had chosen the determination of the nuclear moments of beryllium from the hyperfine spectrum. He developed an atomic beam light source to minimise the Doppler effect, but just before the decisive measurements he was drafted to the air force a few days before the war started. A few months later, having got a leave of absence to finish his thesis, he took his doctoral exam. In 1940, he was exempted from military service and joined again the group around Kopfermann which 2 years later moved to Göttingen, participating in pioneering studies on the hyperfine structure of atomic spectra and - with Wilhelm Walcher - on mass spectrometry and isotopic separation, which was necessary to produce fissionable material for use in making nuclear weapons. In 1944, Paul became Privatdozent at the University of Göttingen.
When Kopfermann heard of the development of the betatron by Donald Kerst in the United States, he immediately saw that scattering experiments with high-energy electrons would enable the study of the charge structure of nuclei. He convinced Paul to turn to this new very promising field of physics.
The betatron was actually the first major machine able to provide high-energy beams of electrons that could be employed in particle physics experiments. The idea to use the electric field around a time-varying magnetic flux for acceleration of electrons that are guided in a magnetic field has many fathers. In 1922, David S. Slepian took a patent on the idea, which was published in 1927, but he never made an experiment. The first, who had in principle all the ingredients in his hands, was the Norwegian engineer Rolf Widerøe. In 1923, as a very young student, he calculated the magnetic field configuration needed to keep the radius of electrons constant during acceleration and found the condition that the field at the orbit must be half of the average field within the orbit. Two years later he realised that in addition the electrons must be stable in the orbit and came to the conclusion that the guide field must decrease with the radius according to a specific power law. He did not publish his results at that time and his published experiments in 1928 were not successful. As an electrical engineer he had no experimental experience with free electrons, but he surprisingly gave the parameters for a 100-MeV accelerator, a challenging dream for that time. In 1935, M. Steenbeck at the Siemens Company also took up the problem of acceleration by magnetic induction. Knowing the Widerøe 1:2 condition, he formulated the magnetic focusing condition for electrons on a stable orbit, that Widerøe had already known. An experimental setup was built, but with extremely low intensity. The first successful induction accelerator was built by Donald Kerst in 1940. Kerst, who had seen Widerøe's publication, was a more careful experimentalist, especially in the magnet design. In a paper with Serber he gave in 1941 the full theory of its operation.
This success of Kerst, published in the last issue of the Physical Review to come to Germany during the war, strongly influenced Paul's personal life, as he himself told later: "At that time I was an assistant to H. Kopfermann, who worked for years on the isotope shifts in atomic spectra, in order to get information about the charge distribution in nuclei. Immediately he realized that scattering experiments with high-energy electrons would be a superior method. He stimulated me to give up spectroscopy and to convert to the new technique of electron accelerators. K. Gund had started also at Siemens a new attempt in constructing for medical purposes an "Elektronenschleuder" (centrifuge), as he called the betatron in those days. In 1943 the energy of 6 MeV was reached, and Gund succeeded in 1947 in extracting an electron beam out of the vacuum tube for the first time. During the same period Widerøe, with R. Kollath and B. Touschek, built in Hamburg a 15-MeV "ray transformer," - that was the name Widerøe used for the betatron - that successfully came into operation in 1944. Fifteen years later Touschek made important contributions to the development of electron storage rings. It is characteristic for the time before the war that the physicists involved in such developments worked completely independently without knowing each other. There was no exchange of ideas or experiences; symposia or special conferences, which we feel today to be indispensable, were almost never held. Nuclear tourism did not yet exist."
When Kopfermann and Paul heard about Gund's project at the Siemens-Reiniger-Werke for a 6-MeV machine, they decided to offer their assistance and were able to conduct first experiments in the spring of 1944 in Erlangen. Together with Rolf Widerøe's 15-MeV betatron, this was the only working machine built during the war in Europe. During the American occupation, in 1945, an order to dismantle the betatron had been issued, but Paul and Kopfermann succeeded in preventing this with the help of the British Military Government. In 1947, they were able to transfer the betatron to Göttingen where they and others used it for several experiments. They also succeeded in extracting the electron beam. But due to the restriction in physics research imposed by the military government, Paul turned for a few years his interest to radiobiology and cancer therapy by electrons in collaboration with his colleague G. Schubert from the medical faculty. He was able to perform some scattering experiments and studied first the electric disintegration of the deuteron; they also measured for the first time the Lamb shift in the He-spectrum with optical methods.
The Siemens betatron has been an exhibit of the Smithsonian Institution Museum in Washington since the 1960s. The development of betatrons for high-energy physics was short, ending in 1950 when Kerst built the world's largest betatron (300 MeV), but they continued to be built commercially for hospitals and small laboratories as they are the very reliable and cheap compact sources of either high energy electrons or X-rays to be used for radiotherapy.
Traps for Charged and Neutral Particles
In 1952, Paul was appointed professor of Experimental Physics at the University of Bonn where he stayed until 1993. There he took over responsibility for the physics institute of the university, whose former directors had included Heinrich Hertz and Heinrich Kayser, but which was in bad shape in the aftermath of the war. Paul and his associates developed research programs on different subjects, ranging from molecular beam physics to mass spectrometry and high-energy electron physics. As he himself recalled in his Nobel Lecture: "It was a scanty period after the war. But in order to become in a few years competitive with the well advanced physics abroad we tried to develop new methods and instruments in all our research." To perform competitive research in such a situation, one had to have new ideas. The best-known results of this research were the electric mass filter, widely used as a residual gas analyser, and the electrodynamic ion trap, which turned out many years later to be one of the essential ingredients for the isolation and detection of single atomic ions.
As Paul explained in his Nobel Lecture, the possibility to observe individual trapped particles opened up a new dimension in atomic measurements. Measurements are generally performed on an ensemble of particles. Therefore, the measured value is averaged over many particles, and tacitly one assumes that all atoms show exactly the same statistical behaviour if one attributes the result to the single atom. On a trapped single atom, however, one can observe its interactions with a radiation field and its own statistical behaviour alone. He also recalled that, “The idea of building traps grew out of molecular beam physics, mass spectrometry and particle accelerator physics I was involved in during the first decade of my career as a physicist more than 30 years ago. In these years (1950 - 55) we had learned that plane electric and magnetic multipole fields are able to focus particles in two dimensions acting on the magnetic or electric dipole moment of the particles. Lenses for atomic and molecular beams were conceived and realised improving considerably the molecular beam method for spectroscopy or for state selection. The lenses found application as well to the ammonia as to the hydrogen maser." In fact, multipole fields were used in molecular-beam physics first, where molecules in the beam can be manipulated by electrical fields and magnetic fields and used to investigate specific properties like the nuclear magnetic moments.
The question “What happens if one injects charged particles, ions or electrons, in such multipole fields” led Paul to the development of the linear quadrupole mass spectrometer. The quadrupole mass spectrometer employs not only the focusing and defocusing forces of a high frequency electric quadrupole field acting on ions - instead of a magnetic field - but also exploits the stability properties of their equations of motion in analogy to the principle of strong focusing - or alternating-gradient focusing - for accelerators which had just been conceived and which immediately attracted Paul's interest. The particle dynamics in such focusing devices is in fact very closely related to that of accelerators or storage rings for nuclear or particle physics. In the principle of strong focusing, the position of the accelerated particles lags behind the alternating gradient, so that they are at defocused position when the field is focusing and vice versa. Being farther from centre, they experience a stronger field when the field is focusing than when it is defocusing. The net effect of a particle beam of charged particles passing through alternating field gradients is to make the beam converge. The theory permitted very high field strengths to be employed, while massively reducing the size of the magnets needed by minimising the size of the beam and was soon applied in the design of new cyclotrons and synchrotrons during the 1950s.
Knowledge of orbit stability in accelerators and extension of the rules of two-dimensional focusing to three dimensions, led Paul to the idea of the quadrupole ion trap, where a confinement potential is created by the radio frequency electric quadrupole field, in order to capture ions for times limited chiefly by collisions with the background gas, but holding them long enough for their properties to be accurately measured. This device consists essentially of three rather oddly shaped electrodes, two of which are virtually identical and, while having hyperboloid geometry, resemble small inverted saucers, called end-cap electrodes. Each has a single small central aperture through which electrons and/or ions can be gated periodically and one also has several small apertures arranged centrally through which ions pass to a detector. The third electrode is also of hyperboloidal geometry and is called the ring electrode, roughly the same size. The ring electrode is positioned symmetrically between two end-cap electrodes. The three components are arranged coaxially about their common axis of rotational symmetry. The geometries of the electrodes are defined so as to produce an ideal quadrupole field that, in turn, will produce a parabolic potential well for the confinement of ions. The potential well is created from the field that exists when a radiofrequency potential is applied to the ring electrode and the two end-cap electrodes are grounded. If one brings ions into the trap, which is easily achieved by ionising a low pressure gas with electrons passing through the volume, they experience in the quadrupole field a strong focusing in that the restoring force, which drives the ions back towards the centre of the device, increases as the ions deviate from the centre of the device. They perform the same forced motions as in the two-dimensional case. The only difference is that the field in the axial direction is stronger. As they perform oscillations in the traps with definite frequencies that at fixed field parameters are determined by the mass of the ion, the quadrupole ion trap enables a mass selective detection of the stored ions. In summary, the ion trap works as ion source and mass spectrometer at the same time. It became the most sensitive mass analyser available, as only a few ions are necessary for detection. Offering very long single-ion storage times and hence a very long period of interrogation, the trapped ions had enormous advantages for high-resolution spectroscopy.
At the same time it was not at all obvious what kind of different applications might result from such a device and for the next 15 years, aside from mass analysis in chemistry, the publications involving ion trap physics were very few.
Promoting Accelerator Physics in Europe
In the meantime, from the mid-1950s on, Paul's interest focused on electron accelerators. He started with a 500-MeV synchrotron, designed and implemented by his team of bright young students and assistants, the first in Europe working according to the new principle of strong focusing. The 500-MeV synchrotron was in use for experiments from 1958 to 1984. Later he built a 2.5-GeV synchrotron in collaboration with H. Ehrenberg, R. H. Althoff and G. Knop, which came into operation for experiments in 1967 and was used for almost three decades as a source of electrons for experiments on the photoproduction of mesons, elastic electron-proton scattering and synchrotron radiation. These machines transformed the institute in Bonn into one of the most important laboratories in Europe. Dozens of students were trained there and transferred their knowledge to many universities, countries and industries.
The technology of strong focusing was suitable to build larger accelerators for high-energy beams. Large accelerators were built at the European Organisation for Nuclear Research, CERN, at Geneva, in Switzerland, founded in 1954, and at the German National Laboratory in Hamburg, started in 1957 by Paul with W. Jentschke and W. Walcher. The experience gained in Bonn was essential in the founding of the new laboratory. The first accelerator built in Hamburg was an electron synchrotron, which delivered an electron beam of 5.3 GeV to experiments. It was called DESY and this name was also chosen for the whole laboratory. Paul was its second director from 1971 to 1973 as well as chairman of its scientific council for several years. The Deutsche Elektronen Synchrotron, DESY, came in operation in 1963. The following higher-energy machines at DESY were the electron-positron colliders DORIS and PETRA and the world-first electron-proton collider, called HERA.
Paul's wide experience in accelerator physics brought him in close contact to CERN, where he spent a year as a guest in 1959, playing a leading role in its early evolution and serving from the very early days as an advisor. Later he became director of the nuclear physics division from 1964 through 1967. The physics Institute in Bonn actually was one of the first external users at CERN and DESY. Paul and his collaborators, as member of an international collaboration, performed a series of experiments with the CERN Proton-Synchrotron and then with the Super Proton Synchrotron. For several more years he acted as chairman of various committees, both at CERN and in Germany, at the same time continuing his research activity on accelerators and participating in the rich experimental program in Bonn. In the early 1990s, when the electron-proton collider HERA became available for experiments at DESY in Hamburg, Paul and his group became member of the ZEUS collaboration, experimenting with head-to-head collisions of electrons or positrons of 28 GeV scattered at protons of 800-900 GeV. Collider experiments at HERA provided data suitable for testing quantum chromodynamics. Another interesting result obtained demonstrates the scale at which electromagnetic and weak nuclear interactions combine into the electroweak force.
Active in research even after retiring, Paul had the idea to extend his trap, which was for charged particles, to neutrons. In this case, sextupole rather than quadrupole fields were needed to act on the magnetic moment of the neutron. He built such a trap, NESTOR, which was installed in a neutron beam at the Institut Laue-Langevin in Grenoble. Paul, and a small team from Bonn which included his two sons Stephan and Lorenz, both physicists, carried out a precision measurement of the lifetime of free propagating neutrons and determined its gravitational mass by seeing the neutrons fall inside the trap. Knowing the exact neutron lifetime is relevant to particle physics, in particular to precision tests of the Standard Model, as well as in astrophysics, because it governs the near equilibrium of protons and neutrons in the primordial phase of nucleosynthesis. The experiment was carried out in 1989 and resulted in the most precise measurement of the lifetime of the neutron at this time.
During the 1970s, the importance of ion traps emerged dramatically when lasers became available as a reliable instrument for spectroscopy of atomic ions. At that time the German physicist Hans Dehmert had been able with his team to trap a single electron. Since his student days in Göttingen immediately after the war, Dehmelt had been interested in the possibility of isolating a single electron, something theorists said couldn't be done. After moving to the University of Washington, he returned to his original interest and he built a device he called a Penning trap, having used an electronic component called a Penning discharge tube. Using this vacuum tube with a strong magnetic field and a weak electric field, throughout the 1960s Dehmelt and his colleagues tried to devise ways of measuring the gyromagnetic ratio, or g-factor, of an electron, which had been calculated theoretically via quantum mechanics. It was in 1973 that they found how to manipulate voltage in order to drive electrons out of the trap until only a single one remained. This breakthrough allowed them to capture and hold single electrons for extended periods of time. After working out a way to cool the trapped electron, slowing its motion and making accurate measurements easier, in 1976 Dehmelt and his co-workers used their trap to observe the quantum jump of a single ion, again confirming a prediction of quantum mechanics. The refinement of these techniques allowed increasingly accurate measurements of the g-factor even for the positron.
The Nobel Prize in Physics 1989 was awarded to Wolfgang Paul and Hans Dehmelt "for the development of the ion trap technique." They shared the Prize with Norman Ramsey "for the invention of the separated oscillatory fields method and its use in the hydrogen maser and other atomic clocks." The technique had in fact as important application the caesium atomic clock and was instrumental in starting the development towards high-precision spectroscopy.
The Nobel Committee acknowledged that "the techniques have reached an unprecedented level of precision, and the development does not yet seem to have culminated." And actually the field has seen a tremendous technical development, as testified by the special volume of Applied Physics B dedicated to ion traps physics on the occasion of the centennial of the birth of Wolfgang Paul. The contributions to the volume clearly hallmark the active ongoing research with Paul traps. The 2012 Nobel Prize in Physics awarded to D. Wineland (together with S. Haroche) "for ground-breaking experimental methods that enable measuring and manipulation of individual quantum systems" clearly recognises the revolutionary development in single-particle spectroscopy and manipulation during the last two decades, which became possible using Paul traps. The ever increasing number of applications continues to have an enormous impact in many fields of physics, especially for atomic clocks, quantum optics and quantum information processing, for accurate mass measurements, the study of chemical reactions at the single-particle level, for cluster physics and for nuclear precision measurements as well as for ion-atom hybrid systems. As the editors of the special volume stress in concluding their editorial note, "Paul traps continue to be an excellent physics tool for decades to come."
Bibliography
Paul E. (2013) 50 Years of experimental particle physics in Bonn. A personal recollection. European Physics Journal H 38: 471-506
Paul W. (1979) Early Days in the Development of Accelerators. In Aesthetics and Science. Proceedings of the International Symposium in Honor of Robert R. Wilson, Batavia, Fermilab, Illinois, April 27, 1979, pp. 25-68
Paul W. (1989) Electromagnetic traps for charged and neutral particles. Nobel Lecture. http://www.nobelprize.org/nobel_prizes/physics/laureates/1989/paul-lecture.html
Paul W. (1989) Biography. http://www.nobelprize.org/nobel_prizes/physics/laureates/1989/paul-bio.html
Toschek P. E. (1994) Wolfgang Paul. Physics Today 47 (7): 76-77
Werth G. et al. (2014) Editorial of Topical issue Frontiers of ion trap and atomic physics: Wolfgang Paul 100. Applied Physics B 114: 1