Prof. Dr. Dorothy Crowfoot Hodgkin > Research Profile
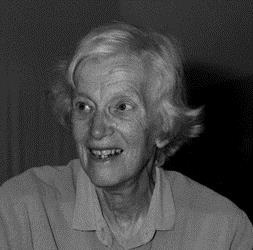
by Luisa Bonolis
Dorothy C. Hodgkin
Nobel Prize in Chemistry 1964 "for her determinations by X-ray techniques of the structures of important biochemical substances".
Dorothy Crowfoot Hodgkin was among the most prominent of a generation of great crystallographers. Beginning in the 1930s, she pioneered the X-ray study of large molecules of biochemical importance, solving a large number of structural problems of importance in biochemistry and medicine. The crystal structures of cholesterol, penicillin, vitamin B12, and insulin are enduring achievements and had an immediate impact on chemistry, biochemistry and medical science.
In 1964, when she was awarded the Nobel Prize in chemistry, she was the first British woman, and the third woman ever, to receive the Prize in chemistry (after Marie Curie and Irène Joliot-Curie). She was the fifth woman to win a Nobel in science and only the second woman to be decorated with the Order of Merit (the first was Florence Nightingale).
Hodgkin reinvented crystallography, transforming it into an indispensable scientific tool. With every major molecule she solved, she devised new methods that expanded crystallography's technical capabilities far beyond what other chemists considered possible at the time. Due largely to Hodgkin's pioneering efforts, X-ray crystallographic techniques were utilized by Max Perutz and John Kendrew in their studies of protein structure, and by Rosalind Franklin, Maurice Wilkins, James Watson and Francis Crick in their analysis of the spiral structure of deoxyribonucleic acid (DNA).
Discovering the Charm of Crystals
Dorothy Mary Crowfoot was born in Cairo, Egypt, of English parents, in 1910. Although her formal schooling took place in England, she spent a significant part of her youth in the Middle East and North Africa, where her father was an inspector for the Ministry of Education. Already at school she was fascinated with chemistry and in particular with crystals, being intrigued by the elegance and beauty of their geometric shapes, with plane faces meeting at characteristic angles, and the orderly symmetry of their structures. She also made small experiments to grow copper sulfate and alum crystals. When she was 14 years old, while visiting her father in Khartoum, where he had just been appointed director of education and antiquities for the Sudan, she met his friend, Dr. A. F. Joseph, a government soil chemist. Joseph further stimulated her interest in science by helping her perform her first quantitative analysis of some local minerals and later giving her a professional surveyor's kit for identifying minerals. Her mother always encouraged her interest in chemistry, allowing her to purchase materials from the local chemist and to do experiments at home.
When Dorothy was 15, her mother gave her two books written by Sir Henry Bragg for school children, where he described how he could shine X rays through a crystal to discover the arrangement of its atoms. Henry Bragg and his twenty-two-year old son Lawrence, along with the German physicist Max von Laue, were pioneers of the new science of X-ray crystallography, the study of the regular packing of atoms and molecules in a crystal. In 1912, Laue had posited that X-rays passing through a crystal would reflect off atomic centres in the lattice and interfere with each other to create a diffraction pattern. Through the 19th and early 20th century, the work of crystallographers on the geometry of crystal structure had shown that the atoms or molecules in a crystal must be arranged in a geometrical pattern with perfect regularity, and it was possible to calculate that the spacing of the pattern must be of the order of 1/100000000 centimetre. The relation between this spacing and the conjectured wavelength of the X-rays was precisely that required to give diffraction effects.
By June 1912, von Laue's idea had been proved right: X-rays, with wavelengths much smaller than interatomic spacings, are able to scatter off the atoms in the crystal, showing the symmetry of the underlying crystal structure. This proved that X-rays behaved like waves, settling a controversy that had lasted the 17 years since their discovery. The points of a space lattice may be arranged in a series of planes, parallel and equidistant from each other. As a pulse passes over each diffracting point, it scatters a wave, and if a number of points are arranged on a plane, the diffracted wavelets will combine together to form a reflected wave front, according to the well-known Huygens construction. In hearing a report of Laue's lecture describing the first observations of the diffraction of X-rays by zinc sulphide, Lawrence Bragg immediately suggested that the diffraction effects could be interpreted as arising from the reflection of X-rays by planes of atoms in the crystal. He realised that analysis of the distribution of high intensity spots that makes up the diffraction pattern can then be used to reveal the underlying symmetry, providing evidence from which the arrangement of atoms in the crystal could be inferred. The pulses reflected by successive planes build up a wave train, which analysis showed to be composed of the wavelengths given by a mathematical equation that allowed Lawrence Bragg to study the structure of a variety of crystals. The simplified calculations that he devised became known as Bragg's Law.
His paper, read by his supervisor J. J. Thomson to the Cambridge Philosophical Society in November 1912, initialized the technique he and his father Henry used for their pioneering work on the structures of minerals, metals, their oxides and alloys. In principle, crystallography is simple. The Braggs beamed X-rays through a crystal and studied the waves that emerged and struck a photographic plate. X-rays and the spaces between the atoms in a crystal are roughly four thousand times smaller than light waves. As X-rays pass between the atoms of the crystal, they are scattered by the electrons surrounding the atoms and are reflected at an angle. Continuing on their separate ways, they strike a photographic plate and form spots. By analysing the relative darkness and lightness of the spots and their positions on the photographic plates, the Braggs could deduce the locations and sizes of the atoms in the crystal. In order to conduct the precise measurements that were required in their investigations, Henry Bragg invented the X-ray spectrometer.
In the 19th and well into the 20th century, chemists conducted long and tedious chemical reactions and degradations to gain clues about the three-dimensional structures of molecules and then performed syntheses to test their deductions. Chemists were examining spectra from spectroscopes not just to analyse for elemental content, but also to gain structural information on molecules. But the examination of crystal structure with the aid of X-rays gave for the first time an insight into the actual arrangement of the atoms in solid bodies. One of the first substances that Lawrence Bragg investigated was sodium chloride, or table salt. At the time, scientists thought that all chemical compounds were made up of molecules composed of atoms. Through his research, Bragg also incontrovertibly established, contrary to what all chemists thought at the time, that sodium chloride in solution was made up of ions, charged atoms of sodium and chloride, and not molecules. This finding added to the scientific understanding of solutions.
The Braggs' approach provided a reliable way to determine the internal architecture of all crystalline solids, and thus to explain their properties. Once the structure of diamond was discovered – with its infinite array of carbon atoms bonded strongly to others in three dimensions – its hardness could be understood. Likewise, when X-ray crystallography revealed the structure of graphite in the 1930s, its softness made sense. Diamond and graphite have the same composition, but their structures make them mechanically, chemically and electronically very different. The structure of diamond confirmed the tetrahedral coordination of carbon as envisaged by J. H. van't Hoff and others 40 years earlier.
At this time X-ray crystallography was still in its infancy. One had to crystallise the substance under study, shoot X-rays at the crystal, and then study the way the X rays were diffracted off the planes of the crystal’s structure. But it soon became an important technique for determining the sizes, shapes, and positions of the atoms and molecules in a crystal. It revolutionised physics and chemistry, and would do the same for biology. Since then, researchers have used diffraction to work out the crystalline structures of increasingly complex molecules, from simple minerals to high-tech materials and biological structures, including viruses. Since the beginnings of modern crystallography in X-ray diffraction experiments on crystals, the discipline has informed almost every branch of science by providing a means to understand the structure of complex molecules and materials.
The collaboration between father and son ended with the outbreak of World War I in 1914. In 1914, von Laue was awarded the Nobel Prize in Physics “for his discovery of the diffraction of X-rays by crystals”, and the following year the Braggs shared the 1915 Nobel Prize in physics “for their services in the analysis of crystal structure by means of X-rays”. At 25, Lawrence Bragg is still the youngest ever recipient of the Nobel Prize.
In reading Henry Bragg's books, Dorothy Crowfoot was particularly fascinated by his description of how X-rays had enabled scientists to “see” individual atoms and molecules. These readings, introducing her to the use of X-ray diffraction to solve chemical structures, definitely influenced her decision to do science. From 1928 to 1932, Crowfoot studied chemistry at Oxford, and, after taking a special course in crystallography, she was encouraged to work at a research project involving the use of X-rays to study the crystal structure of the dimethyl thallium halides.
In 1932 she became only the third woman to achieve a first-class honours degree in chemistry from Oxford. After her successful undergraduate career at Somerville College, in October of that same year she moved to the Department of Mineralogy at Cambridge University as a PhD student, with John Desmond Bernal, a former Bragg collaborator, as her supervisor. Bernal, who was widely known as a brilliant scientist, had just been appointed by Cambridge University to start research in X-ray analysis. Like both the Braggs, Bernal was a firm believer in equal opportunities for women. They turned crystallography into one of the few physical sciences employing significant numbers of women.
Bernal was pioneering the use of X rays to study biological crystals, especially proteins, the most diverse and important chemical constituents of cells. By decoding their molecular structure, he hoped to understand their properties, reactions with other chemicals, and their synthesis from simpler compounds. The Braggs had worked primarily with inorganic molecules while developing their methods; now X-ray diffraction was being used for the first time in conjunction with optical techniques to characterise all sorts of crystals, to deduce molecular masses and to analyse molecular dimensions.
Yet X-ray crystallography was still a relatively new technology with many challenges – and hence opportunities for research – when Hodgkin entered the field. Her PhD project was an X-ray diffraction analysis of crystals of sterols (solid cyclic alcohols, such as cholesterol, found in biological tissues). The idea behind these experiments was that it should be possible to determine the chemical structures of complicated molecules from the relative positions in space of the atoms within them. The diffraction spectra produced when X rays pass through crystals are the consequence of interference between wave trains scattered by the electrons in the atoms and can formally be recombined as in a lens to show the periodic atomic arrangement responsible for their appearance. Henry Bragg pointed out in 1915 that though we have no lens capable of recombining the X-ray waves they could be recombined mathematically by the use of the relations discovered by Fourier, who represented periodic structures by adding together waves of known phase and amplitude. The X-ray spectra correspond with a series of harmonic terms, which can actually be recombined to give a representation of the X-ray scattering material in the crystal, the electron density. The calculation involves the summation of a Fourier series in which the terms have the amplitude and phases of the observed spectra; both depend on the positions of the atoms in the crystal. His son Lawrence Bragg and others made the necessary measurements and calculations on simple crystals of known structure and showed that the atoms appeared as discrete peaks of electron density. At the heart of X-ray crystallography is the electron-density map, generated by the Fourier summation of phased amplitudes, with the individual atoms positioned at its peaks. The chief experimental difficulty in extending the results to crystals of unknown structure involved the experimental determination of the phase relations of the scattered waves (readily calculated for crystals of known structure); the amplitudes were easily measured from the intensities of the diffraction spectra, which had to be estimated by eye.
Bernal's optical and crystallographic investigations had led him to propose a long thin molecule for the sterol skeleton, very different from other proposals. With Bernal, Dorothy investigated sterols using crystallographic methods determining their space groups, unitary cell dimensions and molecular masses. These studies allowed an understanding of the structures of the sterol and of particular compounds, and Bernal’s model was finally accepted by chemists, persuaded by the strengthening crystallographic arguments. All previous chemical formulas turned out to be wrong. Crucial crystallographic results finally led to the derivation of the correct chemical formula for cholesterol.
During the last months of her fellowship at Cambridge, Crowfoot became involved in a project that profoundly influenced her subsequent career. In April 1934, Bernal acquired some crystals of the digestive enzyme pepsin from the University of Uppsala in Sweden. Several crystallographers had already taken X-ray diffraction pictures of dried protein crystals, but obtained no diffraction pattern. The pepsin crystals had been brought to England in their mother liquor, the solution in which they formed. Bernal discovered that air-dried crystals of pepsin exposed to X-rays gave no spots only resulting in a general vague darkening of the film, while crystals suspended in their mother liquor – as the protein would be in the living organism – remained clear and gave sharp diffraction patterns, which indicated that within the pepsin crystals there were very large molecules of definite size and regular arrangement. The regularity of the spots on the X-ray photograph proved unmistakably that proteins can be crystallised and that the arrangement of atoms in crystalline proteins would some day be “seen”. These were the first X-ray photographs of crystalline proteins, providing the first demonstration of atomic regularity in a globular protein. They offered a hope of solving the protein structure; but fulfilment of that hope was still remote because it was hundreds of times more complex than any crystal structure yet solved.
Direct participation in the beginnings of protein crystallography was one of the most important scientific episodes in Dorothy's life. She co-authored with Bernal a paper about the X-ray photographs of crystalline pepsin, published in Nature in 1934 and after two years of challenging and rewarding work in Bernal's stimulating environment, during which she had become a serious scientist, utterly committed to doing research, in September 1934 she returned to Oxford to take up a teaching fellowship at Somerville.
The Insulin Structure
In Oxford she was given the possibility to equip her own X-ray laboratory, where she established an independent research program and continued to work on her PhD thesis, which she successfully defended in 1936. That same year she was awarded a permanent fellowship from Somerville College.
X-ray crystallography was in full bloom in the mid-1930s and new methods were being explored to reconstruct the atomic lattices of the crystals. A series of mathematical computations were needed in order to relate the spots on the photographic plates to the distribution of the atoms within crystals. For even a simple crystal, tens of thousands of calculations had to be done. They produced an electron density map providing indications on the electron-packed regions of the crystals. The density peaks indicated the most likely places to find atoms. Hodgkin developed an incredible skill at interpreting electron density maps: At that time word was already getting around that Hodgkin, like Bernal, had remarkable insight in X-ray photographs. Soon her desk was cluttered with crystals.
Recent studies had shown that proteins contain thousands of atoms. When Crowfoot was offered crystals of the protein hormone insulin, a protein containing fewer atoms than pepsin, she decided that insulin was a suitable molecule for her first independent investigation, seeming a more hopeful candidate for X-ray analysis. This encounter started a 34-year-long journey to its solution. Though insulin was still much too large a molecule to study in detail then, she could not resist growing the crystals and making measurements. To make crystals large enough for X-ray analysis, Crowfoot re-precipitated the insulin in the presence of zinc. After putting the crystal in front of an X-ray beam, she placed a photographic plate behind it, and that same night, when she developed the film, she saw minute, regularly arranged spots forming a diffraction pattern that held the prospect of solving insulin's structure. From the first X-ray photograph of insulin crystals in 1935, Crowfoot was able to conclude that zinc insulin crystals had rhombohedral symmetry, which meant that they possessed a threefold axis of rotation. This showed that the unit cell contained either one molecule that itself had threefold symmetry or 3n molecules, arranged symmetrically but without an internal symmetry of their own. From density measurements, she calculated the weight of protein in the unit cell and, by a more detailed analysis, she could even calculate that such a unit contained about 520 carbon, 130 nitrogen, 150 oxygen, and 12 sulphur atoms, and that there were also present a large number of water molecules in the crystal. However, before setting out to find the atomic positions in such a complicated crystal, it seemed desirable to try direct X-ray analysis on something simpler. Crowfoot published a detailed analysis of zinc insulin in 1938, but at that time she never imagined that it would take her 34 years to solve that complex structure, nor that once solved it really would have medical applications.
Crowfoot performed diffraction on insulin crystals at the Royal Institution, in London, using Henry Bragg's powerful X-ray tube. While in London she met Thomas Lionel Hodgkin, whom she married in December 1937. However, the following year, at age 28, she was diagnosed with a crippling case of rheumatoid arthritis, which deformed her hands and brought her tremendous pain for the remainder of her life.
In the late 1930s she studied lactoglobulin and other proteins for which crystals were available. But after that she set aside protein crystallography for new promising lines of investigation.
A Vital Weapon: Penicillin
During World War II, Bernal's new X-ray equipment, and with it some students and associates, evacuated to Oxford from Birkbeck College, University of London, where Bernal was now a professor of physics. Hodgkin put Bernal's student Harry Carlisle to work on the analysis of the detailed structure of cholesteryl iodide skeleton using as little chemical information as possible. They were able to confirm the molecule's chemical structure and give accurate dimensions and stereochemical configurations. Their approach, leading to the first three-dimensional analysis of a complex organic molecule, demonstrated crystallography's potential for resolving chemical and chiral features. It was the first of Dorothy's crystallographic triumphs and formed the basis of later studies on more complex molecules.
Later in the war, Hodgkin became involved in a project that was deemed to be of significant military importance: an analysis of the structure of penicillin, which again demonstrated the capacity of X-ray analysis to answer chemical questions. This antibiotic, which had been discovered by Alexander Fleming in 1928, was being intensively studied from a chemical point of view. With millions of war casualties anticipated, a drug that prevented bacterial infection was a vital weapon. British and American authorities declared penicillin a “high security secret” and only those working in the field could get research reports. Some of the first penicillin crystals made in the United States were flown to Hodgkin. Late in the summer of 1942, she began a study of penicillin hydrochloride. Penicillin is still a very small molecule compared with insulin, but its chemical structure was essentially unknown. Structural information would be a great aid in the development of methods to synthesise and produce the large quantities needed. At first, penicillin looked hopeless. It was far more difficult to analyse than a sterol. Unbeknownst to anyone, penicillin crystals come in several shapes and Americans and British were working with four different types. Even mild changes in conditions change the way the molecules pack within a penicillin crystal. At that time Hodgkin had only one graduate student, Barbara Rogers Low, to help her on the project. The biggest problem was that no one knew the chemical groups that make up penicillin. Much of what chemists thought they knew turned out to be incorrect. Hodgkin and Low were starting more or less from scratch. In 1943, two different forms of penicillin were crystallised: benzylpenicillin, or penicillin G, and 2-pentenyl penicillin, or penicillin F. Passing X-rays through penicillin crystals from all possible angles, they examined the resulting diffraction patterns recorded on photographic plates and calculated the positions of key atoms in the crystal lattice. Later, use of an IBM card-punch machine facilitated the laborious tasks of plotting the electron-density maps by which Hodgkin and her colleagues determined the molecular structure of penicillin. They discovered that part of the molecule consists of a beta-lactam ring, which later turned out to be part of the core structure of several antibiotic families. Actually all penicillins are made of a five-sided thiazolidine ring attached to a beta-lactam ring. No one had ever seen components joined in such an unusual way.
The three-dimensional structure of penicillin was published in 1949. Penicillin proved that Hodgkin was a master crystallographer. In 1947, at the age of only thirty-seven, she was admitted to the Royal Society of London, the nation's most prestigious scientific organisation. She was only the third woman elected to the 287-year-old society.
The Challenging Complexity of Vitamin B12
In 1948, even before completing work on penicillin, Hodgkin and her colleagues began work on the molecular structure of vitamin B12. The factor that acts against pernicious anemia, a potentially fatal blood condition, was discovered in 1926, but its isolation and purification were achieved only in 1948. When the tiny deep-red crystals of vitamin B12 became available in Oxford, Dorothy was immediately interested in taking on its structure determination, even though very little was still understood about its chemical composition. Chemists needed to know the entire structure before they could hope to manufacture the life-saving vitamin in bulk. Hodgkin knew nothing about the molecule, other than that it was intermediate in size, with roughly 100 non-hydrogen atoms. All this made such analysis an almost hopeless prospect, as the first photographs clearly showed. Vitamin B12 had a structure that had not been seen in nature previously; it had a molecular weight larger than that of any other known vitamin. Dorothy Hodgkin coordinated the collection of data by several researchers for a six-year period. They grew more and larger crystals and took twenty-five hundred X-ray photographs of them. In the beginning, they used the same punch card machine that had been used to determine the structure of penicillin, but soon electronic computers became available to aid in performing the calculation. They were sophisticated enough to handle all the complexities and six years of data and became fundamental in helping to eventually elucidate the molecular structure of vitamin B12, which is actually the most chemically complex of all the vitamins. Hodgkin discovered that the structure of vitamin B12 is based on the corrin ring, an entirely new feature in organic chemistry, now considered one of X-ray crystallography's major contributions to organic chemistry.
Hodgkin could announce the complete structural formula of vitamin B12 eight years after she had started. The final paper was published in 1957. The announcement of the vitamin B12 structure had an enormous impact. It was the largest and most complex organic molecule to have its structure determined in complete detail. Its complexity had put it beyond the capacity of classical approaches of chemical analysis. Moreover, the crystal structure provided a basis for new synthetic approaches. It was now clear that X-ray analysis was the most effective tool for determining structure in the field of natural-product chemistry.
“For her determination by X-ray techniques of the structures of important biochemical substances,” Hodgkin was awarded the 1964 Nobel Prize for Chemistry. In the presentation speech the determination of the structure of penicillin was described as “a magnificent start to a new era of crystallography.” At the same time, the determination of the structure of vitamin B12 was considered “the crowning triumph of X-ray crystallographic analyses, both in respect of the chemical and biological importance of the results and the vast complexity of the structure.”
The Last Word on Insulin Structure
Hodgkin's third major achievement was the determination of the structure of insulin in 1972. It came as a result of more than 30 years of research following the first X-ray photographs in 1935. Insulin contains almost 800 atoms (vitamin B12 is composed of 90 atoms) grouped in 51 amino acids. It was the most complicated structure of her career. This work was further complicated because insulin crystallises in several different forms. Crystallographic studies ran parallel with organic chemical studies ever since the first isolation of insulin crystals, so she got very excited when Frederick Sanger determined the exact ordering in the sequence of amino acids in the molecule. In order to discover insulin's three-dimensional arrangement, she had to analyse seventy thousand X-ray spots, but computer technology had begun to catch up with the complexities of the problems involved and the necessary calculations could be carried out. Skill and imagination did the rest. By late July 1969, Hodgkin's team realised that the insulin electron density map was interpretable.
Hodgkin was able to explain the unique three-dimensional arrangement of the amino acid side groups that lead to hydrogen bonds, disulfide bridges, and energetically favourable structures. The long chains were folded into compact molecules that formed six structural subunits around two zinc atoms. In the Nature article announcing the insulin structure the names of her team members appear alphabetically.
By that time, X-ray crystallography was sometimes the only method for discovering the three-dimensional shape of an organic molecule. One of the traditional aims of organic chemistry – elucidating the molecular constitution of natural substances – was now becoming obsolete. The structure of the molecules involved in the chemistry of life processes are frequently so complicated that attempts to represent them by formulas on paper give little account of the true shape of the molecule and fail to represent important spatial relations. A stage had been reached, where the crystallographer's results needed to be reconciled with data obtained from the accurate amino acid analysis of the proteins concerned. However, as Hodgkin remarked in her Nobel lecture in 1964, one should keep in mind that not all structural problems can be settled by X-ray analysis and that not all crystal structures are easy to solve. She said: “I seem to have spent much more of my life not solving structures than solving them.”
Bibliography
Dodson G. (2002) Dorothy Mary Crowfoot Hodgkin, O.M. 12 May 1910--29 July 1994. Biographical Memoirs of Fellows of the Royal Society 48: 179-219
Ferry G. (1998) Dorothy Hodgkin: A Life. Granta Books, London
Hodgkin D. (1971) X Rays And The Structure Of Insulin. The British Medical Journal 4 (5785): 447-451
Hunter G. K. (2008) Hodgkin, Dorothy Mary Crowfoot. Complete Dictionary of Scientific Biography. Vol. 21. Detroit: Charles Scribner's Sons, pp. 333-339. Gale Virtual Reference Library, http://go.galegroup.com/ps/i.do?
id=GALE%7CCX2830905753&v=2.1&u=mpi_vb&it=r&p=GVRL&sw=w&asid=2c85d89ca7f22c1743d78fbcb0cae511
Hunter N. W. (1993) Doroty Hodgkin. In K. J. Laylin (ed.), Nobel Laureates in Chemistry. 1901-1992. American Chemical Society and the Chemical Heritage Foundation, pp. 456-461
McGrayne S. B. (1993) Dorothy Crowfoot Hodgkin. In Nobel Prize Women in Science. Their Lives, Struggles, and Momentous Discoveries. Carol Publishing Group, pp. 225-254
Wasson T. (ed.) (1987) Hodgkin, Doroty C. In Nobel Prize Winners, H. W. Wilson Company, New York, pp. 470-472