Prof. Dr. Paul J. Crutzen > Research Profile
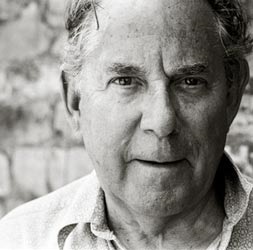
by Luisa Bonolis
Paul Joseph Crutzen
Nobel Prize in Chemistry 1995 together with Mario J. Molina and F. Sherwood Rowland
"for their work in atmospheric chemistry, particularly concerning the formation and decomposition of ozone".
From civil engineering to atmospheric chemistry
Paul Joseph Crutzen was born in Amsterdam in 1933. His mother was of mixed German and Polish origin and his father had relatives in the Netherlands, Germany and Belgium. Thus, from both parents, he inherited a cosmopolitan view of the world. When he was seven, the Netherlands was overrun by the German army and his six years of elementary school largely overlapped with the 2nd World War. He survived the horrible last year of war during which so many children died, and in 1946, after a successful entrance exam, he entered high school to be prepared for University entrance. In 1951, he finished school with natural sciences as his focal subject and with a strong interest in mathematics and physics. He had also become very fluent in 3 foreign languages: French, English and German. Since he could not afford to go to a university, he chose to attend the Middelbare Technische School, middle technical school, to train as a civil engineer, with a 21-month interruption for compulsory military service, during which he worked at the Bridge Construction Bureau of the City of Amsterdam. He graduated with a degree in civil engineering in 1954, and after his marriage with Terttu Soinen, Crutzen settled in a little town north of Stockholm, working in a building construction bureau, but continuing to long for an academic career.
One day, at the beginning of 1958, he saw an advertisement in a Swedish newspaper from the Department of Meteorology of Stockholm University, announcing an opening for a computer programmer. Although he had no experience in the subject, he applied for the job and had the great luck to be chosen from among many candidates. At the beginning of July 1959, he moved to Stockholm and started his second profession.
In the late 1950s, Scandinavian meteorologists were at the forefront of meteorological research. By 1959, researchers from all over the world were coming to the Meteorology Institute of Stockholm University (MISU) and the associated International Meteorological Institute (IMI), which had been founded only about a year earlier by Gustav Rossby, one of the greatest meteorologists ever. The increase in computing power in the 1950s enabled the development of the very first computer models of atmospheric phenomena. In the early 1960s, Stockholm University housed the fastest computers in the world. At that time, programming was a special art. Advanced general computer languages, such as Algol or Fortran, had not been developed, so that all programmes had to be written in specific machine code. Until 1966, Crutzen was mainly involved in the conception, development and running of some of the very first numerical models for weather prediction. He also programmed a model of a tropical cyclone for Hilding Sundquist.
The great advantage of being at a university department was that he got the opportunity to follow some of the lecture courses that were offered at the university. By 1963, he could thus fulfil the requirement for the Filosofie Kandidat (corresponding to a Master of Science) degree, combining the subjects mathematics, mathematical statistics, and meteorology. Unfortunately, he could include neither physics nor chemistry in his formal education, because this would have required his participation in time-consuming laboratory exercises. In this way, he became a pure theoretician, even if he always continued to feel very close to experimental work.
A thesis on the stratospheric ozone
Being employed at the meteorological research institute, it was quite natural to take a meteorological topic for his Filosofie Licentiat thesis. Building on his earlier experience, the further development of a numerical model of a tropical cyclone had been proposed to him. However, around 1965 he was given the task of helping a scientist from the US to develop a numerical model for the distribution of the oxygen allotropes (i.e. its three variants: atomic oxygen, molecular oxygen and ozone) in the stratosphere, the mesosphere and the lower thermosphere. As he recalled in his autobiography written on the occasion of the Nobel Prize, “This project got me highly interested in the photochemistry of atmospheric ozone and I started an intensive study of the scientific literature. This gave me an understanding of the status of scientific knowledge about stratospheric chemistry by the latter half of the 1960s, thus setting the 'initial conditions' for my scientific career. Instead of the initially proposed research project, I preferred research on stratospheric chemistry, which was generously accepted.” Crutzen's career thus took a decisive turn.
At that time, the main topics of research at the Meteorological Institute at the University of Stockholm were dynamics, cloud physics, the carbon cycle, studies of the chemical composition of rainwater, and especially the “acid rain” problem which was largely “discovered” at MISU through the work of Svante Odén and Erik Eriksson. Crutzen’s Filosofie Licentiat thesis “Determination of parameters appearing in the 'dry' and the 'wet' photochemical theories for ozone in the stratosphere,” which discussed the parameters for various photochemical theories regarding ozone in the stratosphere, focused his interest on the ozone layer. The ozone layer is located ten miles above the surface of the Earth and is approximately 20 to 30 miles thick. In the upper atmosphere, the UV wavelengths below 240 nm can be absorbed by O2, splitting it into two oxygen atoms (O2+UV light (<240 nm)-->O+O), thus initiating a sequence of reactions. Each of these O atoms normally adds itself to another O2 molecule to form ozone, O3. Collision with some third molecule, M, is needed to stabilise the O3 product (O+O2+M-->O3+M). These ozone molecules in turn can absorb ultraviolet radiation, with absorption increasing greatly in strength at shorter wavelengths in the UV, and split off an O atom (O3+UV light-->O+O2). Such absorption is especially strong for wavelengths shorter than 290 nanometres. Again, these O atoms usually reform ozone by reacting with O2. The last two rapid reactions lead to the establishment of a steady state relationship between the concentrations of O and O3 without affecting the concentration of odd oxygen. Destruction of odd oxygen, counteracting its production by the already mentioned reaction O2+UV-->O+O, occurs via the reaction O+O3-->O2+O2 because ozone is actually quite chemically reactive and sometimes intercepts the O atoms, forming two O2 molecules. This set of four reactions involving O, O2 and O3 was already recognised in 1930 by Sydney Chapman, who formulated the first photochemical theory for the formation and decomposition of ozone in the atmosphere. Through these processes, a balance of ozone is maintained in the atmosphere by which about 3 parts in 107 of the entire atmosphere are present as O3, versus almost 21% as O2. About 90% of these ozone molecules are present at altitudes between 10 and 50 km, i.e. in the stratosphere, where the mixing ratio of O3 can rise as high as 1 part in 105. The solar UV energy absorbed by O3 is converted into heat, providing a heat source in the 30-50 km altitude range. This heat source creates the stratosphere, the region between 15 and 50 km characterised by an inverted - that is, increasing - temperature profile that rises typically from 210 K at its base at 10-15 km altitude, to roughly 275 K at 50 km altitude.
Because both O2 and O3 can absorb short wavelength UV radiation, no solar radiation with wavelengths < 290 nanometres penetrates below the stratosphere. The ozone layer thus performs two important physical processes: it removes short wavelength UV radiation and changes its energy into heat, maintaining the stratosphere.
That ozone shields the Earth from biologically damaging UV was already known. For nearly a billion years, ozone molecules in the atmosphere have protected life on Earth from the effects of ultraviolet rays. UV-B radiation (280- to 315- nanometre wavelength) from the Sun is partially absorbed in this layer. As a result, the amount of UV-B reaching Earth’s surface is widely reduced, greatly diminishing risks of skin cancer, cataracts, suppressed immune system and possible damage to terrestrial plant life, single cell organisms, and aquatic ecosystems.
Detailed knowledge of the Earth's atmospheric ozone distribution, seasonally and geographically, was outlined beginning in the 1920s by a series of experimenters, especially G. M. B. Dobson, who began regular measurements with a UV spectrometer. Dobson realised that careful measurements of the relative intensities of solar UV radiation at different wavelengths could be converted into quantitative estimates of the amount of ozone overhead, and his initial work near Oxford established that the amount of ozone varied from day to day and month to month. The modern era of stratospheric ozone studies began in the 1950s with proposals that the detailed ozone source and removal processes might not be in balance. Rocket experiments were well underway, and extensive data were now available for the upper reaches of the atmosphere and for outer space. A conclusion began to be formed: less ozone, perhaps by a factor of two, was actually present in the atmosphere than would be expected if the Chapman reactions were the only significant contributors to its creation and removal. Because the formation reactions for ozone seemed solidly based, the “missing” factor was more likely that some additional removal process must exist for ozone. This led scientists to look for other chemical reactions contributing to the reduction of the ozone content. In the mid-1960s, first proposals for the missing sink fell on a free catalytic chain initiated by the highly reactive hydroxyl radical HO in reactions absorbing O and O3 and releasing O2. The ozone destruction reaction pair involving HO and HO2 radicals as catalysts HO+O3-->HO2+O2 and HO2+O3-->HO+2O2 were postulated in 1965 by J. Hampson and incorporated in an atmospheric chemical model by B. J. Hunt the following year. In these reactions, HO reacts catalytically, without itself being consumed. It is able to oxidise most of the chemicals found in the troposphere and therefore is known as the “detergent of the atmosphere.”
In his Filosofie Licentiat thesis of 1968, Crutzen analysed the proposal by Hampson and Hunt and concluded that the rate constants for the two reactions could not explain the vertical distribution of ozone in the photochemically dominated stratosphere above 25 km. Furthermore, he pointed out that the proposed rate constants would also lead to unrealistically rapid loss of ozone (on a timescale of only a few days) in the troposphere. Crutzen also suggested that: “... at least part of the solution of the problem of the ozone distribution might be the introduction of photochemical processes other than those treated here. The influence of nitrogen compounds on the photochemistry of the ozone layer should be investigated.” Unfortunately no measurements of stratospheric nitrogen oxides (NO and NO2) were available to confirm his thoughts about their potential role in stratospheric chemistry.
Further measurements of the rates for these reactions confirmed that Hampson and Hunt’s proposal by itself was clearly insufficient. The discussion had, however, expanded the scientific thinking beyond the Chapman reactions, and had brought free radical reactions into the picture.
Crutzen's new degree, awarded with the highest distinction, opened doors to a fellowship at the Clarendon Laboratory of the University of Oxford, UK, on behalf of the European Space Research Organisation (ESRO), the precursor of ESA. In the summer of 1969, Crutzen joined the Department of Atmospheric Physics and stayed there for a two-year period. The head of the research group, John Houghton, hearing of his idea on the potential role of nitrogen oxides, NOx, handed him a solar spectrum, taken on board a balloon by David Murcray and coworkers of the University of Denver, and indicated to him that it might reveal the presence of HNO3. After some analysis he could derive the approximate amounts of stratospheric nitric acid, HNO3, including a rough idea of its vertical distributions. New published data made him aware of the presence of NOx in the stratosphere as a result of reactions involving OH and HNO3, giving him enough confidence to submit a paper on catalytic ozone destruction by NO and NO2 based on the simple catalytic set of reactions NO+O3-->NO2+O2 and NO2+O-->NO+O2 with net result equivalent to the direct reaction O+O3-->2O2, destroying odd oxygen.
As a result of a series of further developments it became clear that enough NO is produced via the oxidation of nitrous oxide (N2O) to make Crutzen's catalytic set of above reactions the most important ozone loss reactions in the stratosphere in the altitude region between about 25 and 45 km. N2O is a natural product of microbiological processes in soils and waters. A number of anthropogenic activities, such as the application of nitrogen fertilisers in agriculture, also lead to significant N2O emissions. In 1971, it was not yet known that the rate of increase in atmospheric N2O concentrations for the following decades would be about 0.3 % per year. But the discovery of the indirect role of a primarily biospheric product on the chemistry of the ozone layer greatly stimulated interest in bringing biologists and atmospheric scientists together.
Human activities impact on stratospheric ozone
In the fall of 1970, still in Oxford, Crutzen obtained a preprint of a MIT sponsored Study on Critical Environmental Problems (SCEP), which was held in July of that year. This report also considered the potential impact of the introduction of large stratospheric fleets of supersonic aircraft (US: Boeing; Britain/France: Concorde; Soviet Union: Tupolev) and gave Crutzen the first quantitative information on the stratospheric inputs of NOx that would result from these operations at about 20 km altitude, alarmingly close to the ozone layer. By comparing these with the production of NO by the oxidation of nitrous oxide (N2O), commonly known as laughing gas, he realised immediately that we could be faced with a severe global environmental problem. The participants to the MIT conference, however, clearly had not taken any note of Crutzen's paper in which he proposed the important catalytic role of NOx on ozone destruction. Crutzen, however, had quite clear that he had stumbled on a hot topic and thus decided to extend his 1970 study by treating in much more detail the chemistry of the oxides of nitrogen (NO, NO2, NO3, N2O4, N2O5), hydrogen (OH, HO2), and HNO3. It was not an easy task, also because he did not have a formal background in chemistry. He produced extensive model calculations on the vertical distributions of trace gases in the Ox-NOx-HOx-HNOx system in a paper that was published in fall 1971 in the Journal of Geophysical Research. He drew attention to the potential seriousness of the problem of ozone depletion caused by artificial increase of the mixing ratio of the oxides of nitrogen in the stratosphere, estimating the risks of supersonic traffic (SST) in the stratosphere. Unknown to him, a debate on the impact of SST had erupted in the US. Initially, the concern was mainly related to the catalytic ozone destruction by OH and HO2 radicals resulting from the release of H2O in the engine exhausts. However, Harold Johnston, an expert in laboratory kinetics and reaction mechanisms of NOx compounds, immediately realised that the role of NOx in reducing stratospheric ozone had been grossly underestimated. In summer 1971, his paper entitled “Reduction of Stratospheric Ozone by Nitrogen Oxide Catalysts from Supersonic Transport Exhaust” appeared in Science. In the abstract of this paper, Johnston stated that oxides of nitrogen from SST exhaust posed a great threat to the ozone layer and that the projected increase in stratospheric oxides of nitrogen could reduce the ozone shield by about a factor of 2, thus permitting the harsh radiation below 300 nanometres to permeate the lower atmosphere. Crutzen of course fully agreed with Johnston on the potential severe consequences deriving from the potential impact of stratospheric NOx emissions from SST’s for stratospheric ozone and was happy to have support for his own ideas from such an eminent scientist. In July 1971, he returned to the University of Stockholm and devoted himself mainly to studies concerning the impact of NOx releases from SST on stratospheric ozone. In May 1973, he submitted his inaugural dissertation “On the Photochemistry of Ozone in the Stratosphere and Troposphere and Pollution of the Stratosphere by High-Flying Aircraft” to the Faculty of Natural Sciences and was awarded the degree of Doctor of Philosophy with the highest possible distinction, the third time this had ever happened during the history of Stockholm University.
In large part as a result of the proposal by Johnston that NOx emissions from SST could severely harm the ozone layer, major research programs were started, with the aim to study the chemical and meteorological processes that determine the abundance and distribution of ozone in the stratosphere, about which so little was known that the stratosphere was sometimes dubbed the “ignorosphere”. The outcome of these studies was summarised in a publication by the US National Academy of Sciences in 1975: “We recommend that national and international regulatory authorities be alerted to the existence of potentially serious problems arising from growth of future fleets of stratospheric airlines, both subsonic and supersonic. The most clearly established problem is a potential reduction of ozone in the stratosphere, leading to an increase in biologically harmful ultraviolet light at ground level”. The proposed large fleets for SST never materialised, largely for economic reasons; only a few Concordes went into operation. The research programs, however, greatly enhanced knowledge about stratospheric chemistry and confirmed the catalytic role of NOx in stratospheric ozone chemistry. For the first time, the major environmental impact of human activity had been taken into account by the scientific community. From these experiences in the early 1970s, Crutzen derived a clear vision of his role as a researcher: “Although I had started my scientific career with the ambition to do basic research related to natural processes, the experiences of the early 1970s had made it utterly clear to me that human activities had grown so much that they could compete and interfere with natural processes. Since then this has been an important factor in my research efforts.“ Already by the end of 1971 he wrote in an article published in the “The Future of Science Year Book” of the USSR in 1972: “... the upper atmosphere is an important part of our environment. Let us finish by expressing a sincere hope that in the future environmental dangers of new technological development will be recognizable at an early stage. The proposed supersonic air transport is an example of a potential threat to the environment by future human activities. Other serious problems will certainly arise in the increasingly complicated world of tomorrow”.
The problem of Chlorofluorocarbons in the stratosphere
During the early 1970s, some researchers had turned their interest to the potential input of reactive chlorine radicals on stratospheric ozone. The major chemical components of the mid-stratosphere were well known, and their reaction rate constants with atomic Cl had been measured in the laboratory. In the most thorough of these studies, Richard Stolarski and Ralph Cicerone calculated significant ozone depletions if inorganic chlorine were to be present in the stratosphere at a volume mixing ratio of 1 nanomole/mole of air. They were interested in the possible natural release of HCl to the atmosphere from volcanoes, or of man-made chlorine in the exhaust of solid rockets fuels scheduled for propulsion of the space shuttle. Almost all chlorine atoms react with ozone by the reaction Cl+O3-->ClO+O2 with odd oxygen destruction and formation of ClO, another chemically reactive molecule. What happened to ClO at 30 kilometres? Atomic oxygen is especially abundant in the upper stratosphere between 30 and 50 kilometres altitude. The ClO left by the last step is also chemically reactive and reacts with atomic oxygen to re-release atomic chlorine (ClO+O-->Cl+O2), leaving a second molecule of diatomic oxygen. The net result of these catalytic reactions is: O+O3--> 2 O2. This reaction sequence is very similar to the catalytic NOx cycle.
The study by Stolarski and Cicerone, first presented at a conference in Kyoto, Japan, in the fall of 1973, struggled, however, with the problem of a missing chlorine source in the stratosphere, because research had shown that the volcanic source is rather insignificant. In the fall of 1973 and early 1974, Crutzen spent some time looking for potential anthropogenic sources of chlorine in the stratosphere. Initially, his main interest was with DDT and other pesticides. Then, by the beginning of 1974, he read a paper by James Lovelock and coworkers who reported atmospheric measurements of CFCl3 and CCl4 over the Atlantic. Such measurements had been made possible by Lovelock’s invention of the electron capture detector for gas chromatographic analysis, a major advance in the environmental sciences. Lovelock’s paper gave Crutzen the first estimates of the industrial production rates of CF2Cl2 and CFCl3. It also stated that these compounds “are unusually stable chemically and only slightly soluble in water and might therefore persist and accumulate in the atmosphere... The presence of these compounds constitutes no conceivable hazard”. This statement had just aroused his curiosity about the fate of these compounds in the atmosphere when a preprint of a paper by Mario Molina and Sherwood Rowland with the title “Stratospheric Sink for Chlorofluoromethanes - Chlorine Atom Catalyzed Destruction of Ozone” was sent him by the authors.
In January 1972, Rowland, too, had heard about Lovelock's measurements of the atmospheric concentrations of the man-made molecule of chlorofluorocarbon CCl3F. In the early 1970s, CFCs were a triumph of the chemical industry, already in wide and growing usage around the world. Invisible, nontoxic and nonflammable, and engineered to be chemically inert, these synthetic chemicals were known for their considerable stability and thus widely used as near-ideal coolants in refrigerators and air conditioners, and in insulation and as propellants in many aerosol-spray products such as deodorants and hairsprays. CFCs do not readily undergo chemical reactions. Scientists at that time therefore believed that CFCs caused no harmful effects. One of the special advantages cited for this molecule was that it would be an excellent tracer for air mass movements because its chemical inertness would prevent its early removal from the atmosphere. Lovelock thus suggested tracking the CFCs to learn more about the atmosphere. Lovelock's shipboard observations showed the presence of CCl3F in both the northern and southern hemispheres, in quantities roughly comparable to the total amount manufactured up to that date.
In early 1973, in submitting his regular yearly proposal to the AEC, Rowland thus included a predictive study of the atmospheric chemistry of CCl3F: what would eventually happen to the chlorofluorocarbon compounds in the atmosphere? That same year, Mario Molina, who had just completed his Ph. D. work as a laser chemist at the University of California Berkeley, joined Rowland's research group as a postdoctoral research associate.
Rowland and Molina had clear that as synthetic molecules CFCs had been designed to be inert and nonsoluble and were unlikely to be decomposed by common atmospheric processes. But they also realised from laboratory data and chemical insight that the CFCs could not remain inert in the atmosphere forever. After CFC's are released into the atmosphere, they rise slowly and essentially nothing happens in the lower atmosphere. It was their considerable stability that allowed them to waft - one spray at a time - into the upper atmosphere where they would readily absorb ultraviolet light (wavelengths below 220 nanometres) whose absorption usually causes the decomposition of simple atmospheric molecules. All multi-atom compounds are capable of absorbing UV radiation if the wavelength is short enough, and almost all will decompose after absorbing the radiation. CFC molecules in the lower atmosphere are protected against this very energetic UV radiation by O2 and O3 molecules at higher altitudes. The CCl3F molecule was known from laboratory studies to be able to absorb UV radiation at wavelengths < 220 nanometres, but to encounter such solar radiation in the atmosphere the molecule must first drift randomly through the atmosphere to altitudes higher than most of the O2 and O3 molecules - roughly to 25 or 30 km. Because at any given time, only a very small fraction of CFC molecules are found at altitudes of 30 km or higher, the average molecule survives for many decades before it is decomposed by solar UV radiation according to the reactions CCl3F+UV light-->Cl+CCl2F and CCl2F2+UV light-->Cl+CClF2.
In 1974, Rowland and Molina calculated the vertical profile to be expected for CCl3F in the stratosphere. The decomposition rate for this molecule escalates rapidly with increasing altitude in the 20-30 km range so that the estimated average lifetime in the atmosphere was in the range from 40 to 55 years, while for CCl2F2 it was 75 to 150 years. However, once at the top of the atmosphere, the decomposition process is so rapid that a CFC molecule would last at most a few weeks if directly released there.
The answer to their original scientific question was that the eventual fate of the CFC molecules is photodissociation in the mid-stratosphere with the release of atomic chlorine, but on a time scale of many decades. The newly released single chlorine atom, Cl, resulting from decomposition of CFCs, is chemically very reactive. At an altitude of 30 km odd oxygen destruction would take place via the catalytic reaction cycle in which almost all chlorine atoms react with ozone by the reaction Cl+O3-->ClO+O2 forming another chemically reactive molecule ClO. Since the radical Cl atom starts this whole reaction, but is not consumed in the process, it is a catalyst for the ozone destruction reaction. Within three months, they realised that when the catalytic efficiency of about 100,000 ozone molecules removed per chlorine atom is coupled with the yearly release to the atmosphere of about one million tons of CFC's, the original challenging and interesting scientific question, had been converted into a potentially grave global environmental problem involving substantial depletion of the stratospheric ozone layer.
Crutzen immediately understood the great importance of Rowland and Molina's paper and decided to mention it briefly during a presentation on stratospheric ozone to which he had been invited by the Royal Swedish Academy of Sciences in Stockholm. An article appeared within a few days in a Swedish newspaper, which quickly attracted wide international attention and Crutzen was visited by representatives of the German chemical company Hoechst and also by Rowland, who at that time was spending a sabbatical year at the Atomic Energy Agency in Vienna.
Crutzen remained of course highly interested in the topic and by September 1974, about 2 months after the publication of Molina’s and Rowland’s paper, he presented a model analysis of the potential ozone depletion resulting from continued use of the chlorofluorocarbons (CFC’s) which indicated the possibility of up to about 40% ozone depletion near 40 km altitude as a result of continued use of these compounds at 1974 rates. Almost simultaneously, Cicerone and his collaborators published a paper in which they predicted that by 1985-1990, continued use of CFCs at early 1970’s levels could lead to ClOx catalysed ozone destruction of a similar magnitude as the natural sinks of ozone. Following Molina’s and Rowland’s proposal, research on stratospheric chemistry further intensified, now with the emphasis on chlorine compounds.
The advent of the Anthropocene
By the summer of 1974, together with his family, Crutzen moved to Boulder, Colorado, where he assumed two halftime positions, one as a consultant at the Aeronomy Laboratory of the National Oceanic and Atmospheric Administration (NOAA), the other as research scientist at the Upper Atmosphere Project of the National Center for Atmospheric Research (NCAR). For six years, under Crutzen's guidance, the NOAA group directed their considerable experimental skills to studies of stratospheric chemistry also strengthening the relationship between research on atmospheric chemistry and meteorology. In 1977, Crutzen took up the directorship of the Air Quality Division of NCAR and with his University of Colorado colleague John Birks he studied how a nuclear war would affect the planet. During that period, however, his scientific work remained centred on the issue of anthropogenic, chlorine-catalysed ozone destruction. In 1980, Crutzen moved back to Europe and became director of at the Atmospheric Chemistry Department of the Max Planck Institute for Chemistry in Mainz, Germany.
Then, in 1984, British Antarctic Survey scientists Joseph Farman, Brian Gardiner, and Jonathan Shanklin discovered a recurring springtime Antarctic ozone hole. It was about the size of the contiguous United States. On this occasion, earlier data coming from a first satellite designed to study ozone depletion processes launched in 1981 were reprocessed accordingly. All this made the ‘ozone hole’ situation, also mapped by an older satellite Nimbus 7, sufficiently obvious to become a global concern.
Rowland and Molina’s scientific investigations, together with Crutzen's researches and commitment, started an environmental movement that ended with a success story for global cooperation on a worldwide environmental threat. Regulations for the control of CFC emissions had first been introduced during the late 1970s for the use of CFC-11 and CFC-12 as propellant gases for aerosol sprays, but only in the United States, Canada, Sweden and Norway. Companies continued to produce CFCs for other uses. Moreover, there was no urgency by the international community to ban CFCs outright, and researchers were challenged to prove that the observed ozone depletion was well beyond natural variations. The 1985 discovery of the huge ozone hole above Antarctica answered this challenge and that same year a United Nations meeting in Vienna, Austria, had agreed to a convention concerning protection of the stratospheric ozone layer. After a series of rigorous meetings and negotiations, the Montreal Protocol on Substances that Deplete the Ozone Layer was finally agreed upon on 16 September 1987 at the Headquarters of the International Civil Aviation Organization in Montreal. A landmark international environmental treaty was signed by 70 countries agreeing to phase out the production and usage of CFCs and other ozone-depleting chemicals and to eliminate inventories of them. Today, nearly 200 countries are signatories, including the United States.
The further manufacture of CFCs has been banned by the 1992 revisions of the 1987 Montreal Protocol of the United Nations. Atmospheric measurements have confirmed that the Protocol has been very successful in reducing further emissions of these molecules. Recovery of the stratosphere to the ozone conditions of the 1950s will occur slowly over the years because of the long lifetime of the precursor molecules. Nowadays, models suggest that the concentration of chlorine and other ozone-depleting substances in the stratosphere will not return to pre-1980 levels until the middle decades of this century. On the other hand, it appears that the increasing impact of the greenhouse gas warming will delay or even postpone the recovery of ozone levels in some parts of the globe. The ozone layer over the tropics and mid-southern latitudes might not return to pre-1980s levels of ozone for more than a century, if ever.
During the 1990s, Crutzen continued working in stratospheric chemistry and several major
atmospheric science instruments onboard ESA environmental satellites originated in his department at the Max Planck Institute.
In 1995, the Nobel Prize for Chemistry was awarded jointly to Paul J. Crutzen, Mario J. Molina and F. Sherwood Rowland "for their work in atmospheric chemistry, particularly concerning the formation and decomposition of ozone".
Building on his fame, especially enhanced after becoming a Nobel Laureate, Crutzen has become more and more active in the support of solutions to limit or fight global warming and has published polemical essays on the topic. In 2002, in his article “The Geology of Mankind”, he launched the word ’anthropocene’, already coined in the 1980s by ecologist Eugene Stoermer for the current geological epoch, marked by the global effects of human activities on the environment. At that time, Crutzen emphasised the great responsibility lying with scientists and engineers: They should assume the task of guiding society towards environmentally sustainable management during the era of the Anthropocene. According to Crutzen, this would require “appropriate human behaviour at all scales and may well involve internationally accepted, large-scale geo-engineering projects, for instance to ‘optimize’ climate. At this stage, however, we are still largely treading on terra incognita.” During the last ten years the idea that humanity has driven the planet into a new geological epoch has gained momentum to the point that scientists are seriously considering the possibility that the Anthropocene, the period beginning with a growth in the atmospheric concentrations of several greenhouse gases, should be formally recognised as a geological unit within the Geological Time Scale, having the same hierarchical level as the Pleistocene and Holocene epochs.
Bibliography
Crutzen P. J. (1995) Autobiography. Nobelprize.org.
http://www.nobelprize.org/nobel_prizes/chemistry/laureates/1995/crutzen-bio.html
Crutzen P. J. (1995) My Life with O3, NOx and and other YZOx Compounds http://www.nobelprize.org/nobel_prizes/chemistry/laureates/1995/crutzen-lecture.html
Crutzen P. J. (2002, January 3) Geology of Mankind. Nature 415: 23
ESA. History of Europe in space. Paul J. Crutzen: the engineer and the ozone hole. http://www.esa.int/About_Us/Welcome_to_ESA/ESA_history/Paul_J._Crutzen_The_engineer_and_the_ozone_hole
Molina M. (1995) Polar Ozone Depletion. Nobelprize.org. http://www.nobelprize.org/nobel_prizes/chemistry/laureates/1995/molina-lecture.html
Rowland F. S. (2006) Stratospheric ozone depletion. Philosophical Transactions: Biological Sciences 361(1469) Reviews: 769-790
Williams M. et al. (2011) The Anthropocene: a new epoch of geological time? Philosophical Transactions of the Royal Society A 369 (1938), Special Issue, http://rsta.royalsocietypublishing.org/content/369/1938.toc