Prof. Dr. Patrick Maynard Stuart Blackett > Research Profile
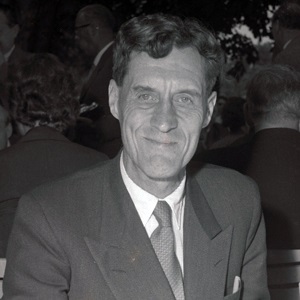
by Luisa Bonolis
Patrick Maynard Stuart Blackett
Nobel Prize in 1948 Physics
Cloud chamber and transmutation of nitrogen
Patrick Blackett was a remarkably versatile physicist, whose career touched science, warfare and politics. His major scientific achievements were in the fields of nuclear physics, cosmic rays, particle physics, and geomagnetism, but his activity as a scientist always went hand in hand with his strong commitment to the development of a healthy science policy in his country. His influence for the benefit of science was of major importance after the end of World War II, being largely responsible for the greatly increased level of support for pure scientific research. As a member of many committees and scientific councils he always exerted a strong influence as an advisor to the British as well as to the Indian governments, because he was convinced that rich countries had to increase aid and technical assistance to less wealthy nations. He strongly supported the development of CERN international laboratory in Geneva, a project on a large scale concerned with the most fundamental problems of physics and involving European collaboration. In 1965, when he became President of the Royal Society, he continued to play a significant part as an adviser to the Government.
Patrick Blackett was born in London in 1897. After attending a preparatory school he studied at Osborne and Dartmouth Royal Naval Colleges in anticipation of a naval career. When World War I broke out in August 1914, Blackett was not yet 17. He participated in battles and war actions on different ships, but before the Armistice he had already resolved to resign from the Navy. A lucky circumstance determined his future. Four hundred of the young officers whose course had been truncated by the outbreak of war were sent to Cambridge for a six-month course of general studies. In this way, Blackett found himself in Magdalene College in January 1919. A few days after arriving there, Blackett wandered into the Cavendish Laboratory, to see what a scientific laboratory was like. At that time, the Cavendish was already becoming a leading centre for the study of radioactivity and atomic structure under Ernst Rutherford. All this sounded so appealing that three months later Blackett resigned from the Navy to become an undergraduate student. He graduated in 1921 with a B.A. in physics. A fellowship enabled him to remain at Cambridge to work under Rutherford on exciting research involving nuclear reactions. In 1919, Rutherford had discovered that by bombarding nitrogen with fast alpha particles from radium, he obtained hydrogen particles of greater energy than could be expected from elastic collisions. It turned out to be the first observation of an artificial nuclear reaction. Rutherford had created “atoms of hydrogen,” which he named protons, with the concomitant production of a new heavier nucleus, a rare oxygen isotope. The discovery of the disintegration of the nitrogen atom was made by observing the scintillations caused by the impact of fast particles on a zinc sulphide screen through a low-power microscope.
When Blackett arrived at the Cavendish, Rutherford continued these experiments on the transmutation of the lighter elements in close collaboration with James Chadwick, using the scintillation method to find out what happened when fast alpha particles hit the nuclei of various light elements. But the technique was slow and limited. Rutherford asked Blackett to become acquainted with and investigate the cloud chamber, a device invented by C. T. R. Wilson, with which tracks of ionising particles could be visualised. Wilson had just brought to perfection such a device, which Rutherford himself considered “the most original and wonderful instrument in scientific history.” Blackett was thus very lucky to begin his scientific career working with this extraordinary tool, which was soon adopted with startling success in all parts of the world and became fundamental for nuclear and particle physics. Many important achievements and discoveries were obtained with the cloud chamber, fully justifying Rutherford's remark. For his invention Wilson was awarded in 1927 the Nobel Prize for Physics.
Rutherford was very impatient to have the instrument perfected for the study of alpha particles bombarding targets. Blackett was able to improve it in order to obtain very sudden expansions and to repeat them as frequently as desired. He perfected a spring action linking the sudden expansion to a camera shutter so that a photograph was taken just as the expansion was completed. Peter Kapitza collaborated with him briefly in developing a powerful magnetic field around the chamber. In 1924, work with the automatic chamber started in earnest and Blackett took 23,000 photographs within a few months. With an average of 18 tracks per photograph, this gave over 400,000 tracks, each of which had to be examined. Eight forked tracks were found which had a quite different appearance from those showing normal elastic collision and these were readily identified as the sought-for transmutation of nitrogen. Photographs showed the capture of an incident alpha particle by a nitrogen nucleus, creating an isotope of oxygen, and the path of a very fast proton ejected from the recoiling oxygen nucleus. This beautiful result provided unambiguous and visible evidence both for the disintegration and for the synthesis of a heavier atom from a lighter, fully confirming Rutherford's expectation that one element can be artificially transmuted into another. It made Blackett's reputation at the age of twenty-seven.
Particles taking their own photographs
At this time, Blackett decided to spend the academic year 1924-1925 in Germany, to study with James Franck at the University of Göttingen. Blackett was among the first of his country's scientists to reopen contacts with Germany, at a time when official relations within the European scientific community were still tense in the aftermath of World War I, and policies of ostracism were still advocated against the Central Powers. His time in Germany not only helped him widen his knowledge of physics but also brought him in contact with the newly developing quantum physics. Blackett returned to Germany in 1930 for a summer in Berlin. By that time he had become one of the leading young physicists at Cavendish, known for his skill in the experimenter's art, combined with a deep theoretical insight. In Berlin, he met Bruno Rossi, a young Italian physicist, who had devised a coincidence circuit integrating electronic valves and Geiger-Müller counters with which he was detecting and investigating the nature and behaviour of cosmic radiation.
During the 1920s, the U.S. physicist and Nobel Laureate Robert Millikan had provided theoretical justifications for his theory according to which cosmic rays were the ‘‘birth cry’’ of atoms in space, i.e., that they were gamma rays from the energy set free in the synthesis of heavier atoms through fusion of primeval hydrogen atoms. As cosmic radiation had a penetrating power far exceeding that of the gamma rays emitted by radioactive substances, it was expected that cosmic gamma-rays traveling through matter would be accompanied by a flow of ‘‘soft’’ secondary electrons resulting from the Compton effect, which were presumed to be the ionizing agent recorded by the measuring instruments. However, a pioneering experiment carried out in Germany by Bothe and Kolhörster during the period 1928-1929 had clarified that this could not be the case. By recording the coincidences between two superimposed Geiger-Müller counters, they had been able to show that the radiation traversing the two counters within a fixed time could not have the nature of a secondary radiation resulting from the Compton effect generated by the ultra-gamma-radiation. Following their experiment, Rossi had perfected the coincidence method, beginning to investigate the nature and behaviour of the cosmic radiation. Rossi’s relevant investigations performed during the period 1931-1932 pointed to the existence of two components in cosmic rays at sea level: a ‘‘hard’’ component, able to pass through 1m of lead after being filtered through a 10 cm thick lead screen, and a ‘‘soft’’ component, generated in the atmosphere by primary cosmic rays and able to generate groups of particles in a metal screen before being stopped. Other absorption measurements on cosmic ray particles had shown the existence of these two components; however, Rossi’s experiments were the first to prove that the hard and soft rays were fundamentally different in character and did not differ merely in their energy. He concluded that it was the soft component that generated groups of particles when traversing metal screens before being stopped.
Rossi suggested that his doctoral student Giuseppe Occhialini join Blackett in Cambridge to learn cloud-chamber techniques.
In 1932, when Occhialini arrived in Cambridge, Blackett's personal research work underwent a great change. Occhialini brought with him the technique of the coincidence counting of cosmic rays developed by Rossi. The marriage of this technique with the cloud chamber was a successful cross-fertilization. Blackett began to use the counter-controlled cloud chamber to study cosmic rays instead of alpha particles. The topic of cosmic rays had already attracted Blackett's attention after Dmitry Skobelzyn published a paper in 1929, reporting cloud-chamber tracks of charged particles associated with cosmic rays. At that time the expansion was made at random and useful tracks occurred only for a small number of expansions. Blackett and Occhialini placed one Geiger-Müller counter above the chamber and another below arranged in a coincidence circuit so that only particles passing through both counters, and therefore lying in the plane of the chamber, triggered the expansion, making particles take their own photographs. The chamber was placed in a magnetic field and the first photographs obtained as soon as they first ran the chamber showed particles on each plate. In early February 1933, a few months after the first announcement, Rutherford communicated to the Royal Society their results showing evidence for showers of particles appearing in their photographic plates as diverging from some region in the material surrounding the chamber. They also acknowledged that the abundant production of secondary particles in the metal screens they were now observing was now confirming the observations made by Rossi with his coincidence circuit.
In their paper Blackett and Occhialini discussed the possible mechanisms for the production of the bifurcating tracks of negative and positive particles appearing in the showers. They were the first to expound the pair formation process, the process underlying the formation of electromagnetic showers, one of the most striking facts of the phenomenology related to cosmic rays. They explicitly linked the pair formation to Dirac’s theory of the relativistic electron, placing it into a theoretical perspective. However, in the meantime, Anderson had already published his conclusions about similar tracks generated by cosmic rays in his chamber. They must be due to “a positively-charged particle comparable in mass and magnitude of charge with an electron.” Soon after, Anderson published his famous photo of the track of an antielectron that he named “positron”. Antimatter had officially entered the realm of physics. Only starting from 1934, with the Bethe-Heitler's landmark paper “On the stopping of fast particles and on the creation of positive electrons,” discussing in detail the energy loss of charged particles passing through matter based on the Dirac equation, views on cosmic-ray phenomena really began to change.
Occhialini had come for three weeks: he stayed for three years. Together with James Chadwick, who had just demonstrated the existence of the neutron - an achievement for which he would be awarded the 1935 Nobel Prize for Physics - Blackett and Occhialini studied the production of positive electrons. Although they immediately received nominations, it was Anderson who in 1936 was awarded the Nobel Prize for Physics for his discovery of the positron. He shared it with Victor Hess, who had established the existence of cosmic radiation at the beginning of the 1910s.
Cosmic rays
After some investigations on the positrons produced by gamma rays from radioactive substances, Blackett's research now became wholly concentrated on cosmic rays, a field that was becoming very promising after the discovery of the positron and that more and more attracted the attention of theoreticians, who were working on the development of quantum electrodynamics.
In 1933, Blackett moved to Birkbeck College in London and one of his first priorities was the construction of a big electromagnet that would amplify deviations in the tracks of charged particles. He also formed an international group including Occhialini and other physicists from Switzerland, India and Singapore.
Studies of the nature of cosmic rays were also coming from quite different investigations. If primary cosmic rays were charged particles - as the above-mentioned results had implied - they would also be affected by the geomagnetic field before entering into the terrestrial atmosphere. A latitude effect was expected indicating a lower intensity of cosmic rays near the equator, where the horizontal component of the geomagnetic field is stronger. In 1930, Rossi had also conjectured the existence of an East-West effect, a second geomagnetic effect which would be revealed by an asymmetry of the cosmic-ray intensity with respect to the plane of the geomagnetic meridian, with more particles coming from East or West, depending on whether the particle charge was negative or positive. By 1933-1934 a series of worldwide experiments definitely confirmed the existence of both geomagnetic effects, proving the corpuscular nature of cosmic rays, and also revealing that they were mostly positively charged. However, the complex nature and the mechanisms underlying the whole phenomenon of ‘‘cosmic rays’’ were far from being clarified.
During the mid and late 1930s, Blackett’s research groups gathered additional evidence for the cosmic-ray cascade or shower effect. Blackett was puzzled by some aspects of the energy spectrum of cosmic rays related to the energy loss of particles in metal plates. In that period, the interaction between cosmic rays and matter was far from being well understood. As many theoreticians and experimenters working in the field, Blackett felt that the existing theory was as yet unable to treat successfully the behaviour of photons and particles of very high energy interacting with matter. Quantum electrodynamics appeared to break down at the high energies involved in cosmic rays.
A theory providing a natural and simple explanation on the basis of the quantum electrodynamics cross-sections calculated by Bethe and Heitler appeared in early 1937 in simultaneous papers by Franklin Carlson and Robert Oppenheimer, and by Homi Bhabha and Walter Heitler. The explanation of the phenomenon of showers induced by cosmic rays in matter (discovered by Bruno Rossi and made visible by Blackett and Occhialini) was an unequivocal success for quantum electrodynamics. It settled for the moment the question regarding the soft component found in the atmosphere, able to generate groups of particles in a metal screen before being stopped, but it left completely unsolved the problem of the much more penetrating component of cosmic rays found at sea level.
In the meantime, Anderson and Seth Neddermeyer in the United States had also been engaged in similar measurements of the energy loss of cosmic ray particles using a cloud chamber of the Blackett-Occhialini type. They had identified in their cloud-chamber photographs a particle that they called mesotron, or heavy electron, because it appeared to have a masse intermediate between that of an electron and that of a proton. After the formulation of the cascade theory and the evidence produced by Anderson and Neddermeyer most physicists accepted the idea of the existence of a brand-new particle, much more penetrating, and probably heavier, than the electron. Robert Serber and Robert Oppenheimer suggested that it was the theoretical particle predicted by Hideki Yukawa in 1935 as a mediator of the short-range nuclear forces. From an analogy with the well-established beta-decay process of radioactive substances, it was assumed that cosmic-ray mesotrons should be unstable, and disintegrate spontaneously into an electron and a neutrino. Cosmic ray physicists were now facing the new challenge of investigating the nature of the mesotron and its decay process. Particle physics was taking shape, emerging as a new field from the confluence of nuclear physics, cosmic-ray studies and quantum field theory.
The mesotron decay
In late 1937, Blackett succeeded Lawrence Bragg in the physics chair at Manchester, a position that previously had been held by Rutherford. Under Bragg the research in the department had been almost entirely concentrated on X-ray crystallography. Within a few months after Blackett's arrival, a great change occurred and everything was organised for the installation of the big magnet and cloud chamber that arrived from Birkbeck in 1938. With remarkable speed, Blackett created a major research centre for cosmic rays in the department and formed a group working on cosmic rays. He found there the young Bernard Lovell and George Rochester, and he brought with him from Birkbeck Lajos Jánossy and J. G. Wilson. Among the many distinguished visiting scientists he had in the department, there were some refugees from Europe. In December 1938, Bruno Rossi arrived from Copenhagen, after having left Italy, where he had lost his chair in Padua because of the fascist racial laws. His presence was instrumental in triggering a large-scale project on cosmic ray research. Rossi and Blackett began to investigate the photon component and the cascade theory. Both had a deep grasp of the essential theoretical concepts and of experimental techniques, which they soon conveyed in two articles appearing one after the other in the December 3 issue of Nature. Drawing upon a theoretical paper by Werner Heisenberg and H. Euler, they discussed the hot issue of the instability of the mesotron - for which only very indirect proofs existed - and calculated its decay times.
In the meantime the situation in Europe was becoming more and more ominous. Following the Anschluss of Austria in 1938 and the conquest of Czechoslovakia in March 1939, Hitler's plans were now revealing their true nature. In June, Rossi and his wife left England, as suggested by Blackett, who feared that they might become enemy aliens, now that Mussolini and Hitler had become firm allies and the war was certainly going to break out. Blackett and Rossi had both been invited to Chicago, where the first major international conference on cosmic rays took place from 27 to 30 June 1939. They presented a paper on some of the recent work on cosmic rays performed in Manchester. Blackett's last publication on cosmic rays before the outbreak of war was again on the instability of the mesotron.
The Invasion of Poland by Hitler's troops that same September marked the beginning of World War II. Rossi, like many European scientists and other refugees, decided to remain in the United States. The participants at the Chicago meeting had agreed that there was no conclusive experimental evidence for mesotron decay. Now, for the first time, physicists were dealing with the phenomenon of spontaneous instability of an elementary particle. Establishing the reality of the decay process and the accurate determination of the mesotron’s mean life had become one of the outstanding problems of cosmic-ray research. In a series of experiments performed with different collaborators and different techniques during the period 1939-1942, Rossi gave definitive proof of the mesotron decay, demonstrating the relativistic dilation of the lifetime of mesotrons in flight, and culminating in the first precise measurement of the mean life of mesotrons at rest. In 1943, Rossi was invited to participate in the Manhattan Project, and until the end of 1945 he worked in the secret laboratory of Los Alamos with many other prominent physicists on solving the unprecedented challenge of constructing a nuclear weapon under the menace of Hitler's Germany.
Operational research during World War II
Already before the beginning of the war, Blackett had joined the Committee for the Scientific Survey of Air Defense that advised the Air Ministry to give high priority to the development of radar for defence against future air attack. At the outbreak of the war, Blackett became deeply involved in war research aiming at improving British antisubmarine warfare and became an expert in the organisation and methodology of operational research. He also led a group that brought about significant improvement in the use of airborne radar for finding German submarines that were sinking merchant ships in the Atlantic. This work was instrumental when U.S. troops reached England for the invasion of Europe during the summer of 1943. However, Blackett was against large-scale bombing of German cities as a means of undermining German morale. Like other scientists, notably John Bernal, he disputed that British citizens' morale had been undermined following the German bombings of British towns. After the war, Blackett wrote the book The Military and Political Consequences of Atomic Energy, where he openly criticised the large-scale bombing strategy, which in his opinion had escalated from the incendiary bombing of Hamburg and Dresden to the nuclear bombing of Hiroshima and Nagasaki. He also interpreted this last decision more as a sign towards Soviet ambitions in Asia and Europe than as arising from the conviction that Japan would not otherwise surrender, arguing that it was intended “as the first act of the cold diplomatic war with Russia”. He also opposed the development of atomic weapons in Great Britain. Because of these views, he was considered by his opponents as a Soviet sympathiser, and for this reason he was not given a visa to travel to the United States to participate in scientific conferences during the 1950s. Since the last months of the war, he became deeply involved in the planning of the post-war development of science, an activity that he pursued with great energy in parallel with his commitment as a teacher and a researcher. He always continued to advocate the social responsibility of the scientist and strong government support of science.
Strange particles
After the war, Blackett reorganised laboratory work at Manchester and succeeded in enlarging his cosmic ray group, which began to work on a very large-scale project of cosmic ray research. With the discovery of the existence of very high-energy particles in extensive atmospheric showers, astrophysical aspects started to become relevant to the question of the origin of cosmic rays. At the same time, cosmic ray physicists continued to play an important role in the field of sub-nuclear physics, since cosmic rays were still the main source of high energy particles. In autumn 1946, George Rochester and Clifford Butler, two young physicists of Blackett's team, discovered two peculiar forked traces in cloud chamber photographs. Blackett was very excited and immediately recognised it as a new phenomenon. Measurements showed that the arms of the V were the tracks of positive and negative particles of large mass, excluding an electron-positron pair. Blackett provided some of the key arguments that convinced Rochester and Butler that what had been called V-particles - having a mass of about 1000 times the mass of an electron - were in fact new neutral particles decaying to two charged particles. A second even more convincing example was found. This time it was a positive unstable particle decaying to one charged particle and one or more neutral particles. As recalled by Blackett's biographer, M. J. Nye, “such was the generosity and integrity of the man, however, that he never sought to include his name in the letter to Nature announcing the discoveries.”
These examples opened up the enormous field of “strange particle physics” at a time when the discovery of the pion decay announced in 1947 by Cecil Powell, Cesare Lattes, Hugh Muirhead and Occhialini at Bristol was clarifying that the mesotron, renamed mu-meson or muon, was actually the decay product of the nuclear force carrier, the pion. The real nature of the muon had already been revealed during the war by the Italian team formed by Marcello Conversi, Ettore Pancini and Oreste Piccioni. In demonstrating that the mesotron was not the Yukawa particle because it did not interact strongly with nuclear matter, this landmark experiment had inaugurated the modern era of particle physics, as later Luis Alvarez remarked in his Nobel Lecture.
In 1949, Rochester and Butler moved the equipment and the magnet chamber to the Pic du Midi, a mountain in the French Pyrenees. New particles were discovered by their team and by other physicists, which added to the growing zoo of elementary particles. These were the last important contributions that cosmic ray physicists gave to the field, before the advent of new powerful accelerators in the early 1950s, which provided the possibility of quantitative systematic measurements at energies which since then have been pushed to higher and higher values.
In 1948, when these important discoveries were starting to have a relevant impact within the physics community, Blackett was awarded the Nobel Prize for Physics “for his development of the Wilson cloud chamber method, and his discoveries therewith in the fields of nuclear physics and cosmic radiation.” In his Nobel Lecture, he reviewed the last 24 years of experimental researches in which he had been occupied as a physicist. Starting with Wilson, to whom he felt indebted more than he could express, he duly mentioned all the people that in some way had been involved in those developments, providing a valuable reconstruction of the history of the use of the cloud chamber for investigating the interaction processes of the sub-atomic particles. As recalled by Mary Joe Nye, “Slightly damping Blackett's euphoria was the disappointment that his dear friend Beppo (Giuseppe Occhialini) was not sharing the Prize.” After a short period in Italy, Occhialini had moved in 1937 to the University of Sao Paulo in Brazil and then to Rio de Janeiro. Only in 1945 was he able to get back to England where he began to collaborate at Bristol with Cecil Powell in the work on mesons that resulted in the announcement of the discovery of the pion in 1947. Occhialini eventually returned to Italy in 1950 and from the early 1960s he led a group working on space physics in Milan. When the Nobel Prize to Powell was announced in 1950, Bruno Pontecorvo was said to have made a famous toast: “I drink not to Beppo, but to us all: may we collaborate with him, it is a practically sure way of winning a Nobel Prize!”
Extensive Air Showers and Cherenkov light
Since the late 1940s, the discovery of particles having energies up to 1015 eV in atmospheric showers led to the investigation of the mechanisms underlying the attainment of such very high energies, which no man made accelerator could ever reach. The cosmic origin of the particles entering the Earth atmosphere could now be investigated, also thanks to the possibility of exploring the high atmosphere with airplanes and rockets provided by war technology. Cosmic ray physics definitely began to assume a more astrophysical character, transforming into a tool for the study of the cosmos. Cosmic rays, which up to that time had provided a natural source of nuclear reactions for investigating the subatomic realm, were now viewed as particles coming from space after having interacted with magnetic fields and space matter, sometimes being endowed with incredible energies. They became tools to investigate phenomena connected to astrophysical objects and fundamental questions related to the birth and evolution of the universe. After the advent of the space age in the late 1950s, cosmic ray physicists were the first to exploit the possibilities coming from space vehicles on which they put their detectors, discovering the van Allen belts, demonstrating the existence of the solar wind, and opening new windows on the universe with the beginning of X- and gamma-ray astronomy.
Blackett, too, was quite interested in the extensive showers of particles generated in the Earth’s atmosphere by primary cosmic rays. In July 1947, he presented the results of his investigations on the possibility of detecting the Cherenkov radiation emitted from fast cosmic ray particles traversing the atmosphere, which opened an entirely new field of cosmic ray investigations.
In the early 1930s, Pavel Cherenkov had observed that when fast electrons traverse a transparent material a continuous spectrum of visible light is emitted in the direction of motion of the particles. The explanation of this phenomenon given by Sergei Vavilov and by Igor Tamm and Ilya Frank clarified that for velocities lower than the phase velocity of light in the medium the phenomenon would not be observed. Blackett suggested that the same effect should be observable in a gas, provided the velocity of the particle approached that of light and he calculated the Cherenkov flux at sea level for cosmic rays traversing the atmosphere by using the theory of Tamm and Frank. He reached the conclusion that extensive air showers should produce a flash of light that could be observed under suitable conditions. His speculations stimulated further investigations, and in 1952 William Galbraith and John Jelley were able to record the Cherenkov light from cosmic rays with a simple apparatus consisting of a 10-inch parabolic mirror with a photo-multiplier at the focus.
In 1958, Cherenkov, Frank and Tamm were awarded the Nobel Prize in Physics for the discovery and interpretation of the Cherenkov effect. Vavilov had unfortunately died in 1951, and Nobel Prizes are not given to dead persons. Since then, Cherenkov emission became a fundamental tool for detecting the passage of particles; techniques based on the effect are commonly used today also in astrophysics experiments, for the detection of high energy cosmic ray showers, in very large neutrino detectors, and in cosmic gamma-ray telescopes, revealing radiation produced by pulsars or other astrophysical objects.
Exploring new fields
Since the wartime, Blackett had discussed with his collaborator Lovell some effects related to the possible connection between the ionisation produced by extremely energetic cosmic ray showers in the atmosphere and occasional sporadic and short-lived echoes on airborne radars. Blackett himself made some experiments to investigate the echo effect. When the war ended, some army radar equipment was acquired and in order to find a site far from the noise of the centre of Manchester, Lovell settled at a place called Jodrell Bank, in Cheshire, where the Botany Department had some land. The initial impetus was the attempt to detect the ionisation due to large showers by radar. The first observations were made in the middle of December 1945, and many echoes were received. It soon became apparent that they were not due to cosmic ray particles entering the atmosphere, but were instead echoes from the plasma trail of a meteor as it burns up in the atmosphere. War-surplus radar equipment for detecting cosmic-ray showers was installed at Jodrell Bank, which quickly became part of the tools for the new field of radio astronomy. In the next few years, supported by Blackett's great determination in finding the funds, Lovell and his expanding team were able to build up the Jodrell Bank Experimental Station that by 1947 hosted the largest radio telescope in the world at that time.
During his investigations on the effect of the sun's magnetic field on cascade showers of electrons, Blackett noticed that the ratio of magnetic moment to angular momentum has a similar value for both the earth and the sun. His speculations on a possible relation between rotation and magnetisation led him to perform a series of experiments using a magnetometer that he himself had designed to detect minuscule magnetic effects. Experiments failed to confirm his ideas about a rotational theory of magnetism, but the magnetometer turned out to be an excellent tool for investigating paleomagnetism in sedimentary rocks. This new research program on the study of rock magnetism played an important part in the modern developments of geological theory, providing a new kind of evidence for Alfred Wegener's 1912 hypothesis of continental drift. About 300 million years ago, claimed Wegener, the continents had formed a single mass called Pangaea that had rifted, or split. Its pieces had been moving away from each other ever since. Wegener was not the first to suggest that the continents had once been connected, but he was the first to present extensive evidence from several fields, such as the close fit between the coastlines of Africa and South America, the large-scale geological features on separated continents matching very closely, and indications by fossils. Reactions to Wegener's theory, which he outlined in the volume The Origin of Continents and Oceans published in 1915, were almost uniformly hostile. Blackett's experiments convinced many sceptics that continental drift was a conjecture that could be tested.
Blackett's interest in rock magnetism continued after he moved to Imperial College, London, in 1953. He created there another great research school, also thanks to the considerable number of staff and technicians whom he was able to transfer from Manchester, achieving continuity of his major research interests. Butler took charge of nuclear physics, Elliot of cosmic rays, and Clegg followed the line of magnetic researches. Under Blackett’s leadership, Imperial College became a top-class Institute for applied and fundamental research. At that time, rock magnetism had become Blackett's own major research interest. Over the next ten years, he stimulated major investigations involving the collection and measurements of samples from different geographic areas and initiated a research project to make a comprehensive palaeomagnetic survey of the whole Indian sub-continent. His group's researches on paleomagnetism and his enthusiastic support of empirical studies of paleolatitudes and paleoclimates, played a huge role in the revival of Wegener's theory. Blackett's magnetometer and its successor instruments were used to gather data that converged in the 1960s with further evidence, leading to an explanation of the continents' past and present motions. A world-wide array of seismometers installed to monitor nuclear testing revealed the astonishing existence of belts defining the edges of tectonic plates along which are aligned earthquakes, volcanoes and other active geologic features. Further paleomagnetic studies revealed that the Earth had undergone several reversals of the magnetic poles in the past, which were recorded in the crust of the ocean basins. This was a positive proof that the lithosphere had to be in motion. Moreover, marine geology developed after the war led to the discovery of the subduction process under the continental margins, a process balancing the extension observed at the mid-ocean ridges by recycling oceanic lithosphere in the mantle. Investigations inaugurated by Blackett's team had provided new fundamental pieces of the puzzle that led to the development of modern plate tectonic theory as an accepted model of geological processes.
Bibliography
Blackett P. (1948) Cloud chamber researches in nuclear physics and cosmic radiation. Nobel Lecture, \url{http://www.nobelprize.org/nobel_prizes/physics/laureates/1948/blackett-lecture.html}
Butler C. Recollections of Patrick Blackett, 1945–1970 (1999). Notes and Records of the Royal Society of London 53: 143-156.
Lovell B. (1976) P. M. S. Blackett. A biographical memoir, The Royal Society, London.
Nye M. J. (2004) Blackett. Physics, War, and Politics in the Twentieth Century, Harvard University Press
Wasson T. (ed.) (1987) Blackett, P. M. S. In Nobel Prize Winners, H. W. Wilson Company, New York, pp. 100-102